Navigating Sustainable Skies: Challenges and Strategies for Greener Aviation
This report outlines challenges, strategies, and policy efforts for greener aviation, both in the United States and globally.
Abstract
Aviation, crucial for global connectivity, significantly contributes to climate change, accounting for 2.5 percent of human-induced CO2 emissions and 3.5 percent of overall human-made changes to the energy balance in the Earth’s atmosphere. The sector’s CO2 emissions have doubled since the mid-1980s, with projections indicating a potential tripling of demand by 2050, underscoring the urgency of greener solutions to balance economic benefits with environmental impacts. However, challenges abound, such as the high costs and sustainability concerns of sustainable aviation fuel, the limited capabilities of alternative technologies, such as hydrogen and electric aircraft, and the need for extensive infrastructure and international collaboration. The report offers an overview of different alternatives for greener aviation and the associated challenges, discusses policy approaches, and highlights areas for future research to effectively reduce the sector’s environmental footprint.
Executive Summary
This report offers an overview of sustainable aviation, focusing primarily on commercial aviation while also addressing a wide range of other aviation operations. It outlines challenges, strategies, and policy efforts for greener aviation in the United States and globally. Importantly, it identifies key research areas for policy development, serving as an introductory guide for researchers interested in aviation sustainability.
Environmental Impacts of the Aviation Industry
Commercial aviation is estimated to account for about 3.5 percent of the overall human-made changes to the energy balance in the Earth’s atmosphere. One-third of this impact is estimated to come from CO2 emissions and the rest from non-CO2 factors, such as nitrogen oxides (NOx) and contrails—the long, thin clouds that often form behind an airplane in cold and humid conditions that trap atmospheric heat. Alarmingly, CO2 emissions from aviation have doubled since the mid-1980s and could account for a quarter of the CO2 budget to limit warming to 1.5°C by 2050. Near airports, aircraft operations elevate pollutants, such as NOx and ozone, adversely affecting air quality and human health. Moreover, airport operations and expansions often increase noise pollution in nearby neighborhoods. Finally, lead emissions from older, small piston-engine aircraft used in smaller non-commercial operations are the largest lead source in US air and present significant health risks.
Challenges
Scaling sustainable aviation technologies: In conventional aircraft, fuel economy can be improved through technological means, such as aerodynamic design, advanced engines, and weight reduction. Airline operations also contribute to efficiency through optimized flight routes, altitude and speed management, and reduced runway idling. However, despite these improvements, emissions from conventional aircraft are rising due to increasing air traffic.
In the near to medium term, sustainable aviation fuel (SAF) offers a viable greener alternative to traditional jet fuel. It is compatible with current aircraft and can reduce greenhouse gas emissions by up to 100 percent and contrail formations by 50–70 percent while also improving air quality compared to traditional jet fuel. SAF is produced from various raw materials, such as fats, oils, sugars, municipal waste, and captured CO2, employing distinct pathways such as hydroprocessed esters and fatty acids (HEFA), Fischer-Tropsch (FT), alcohol-to-jet (ATJ), and power-to-liquid (PtL). Despite this diversity, SAF production currently falls short of global demand. A major challenge for agricultural feedstock is indirect land use change (ILUC), with concerns of deforestation and habitat destruction associated with cultivation. Edible crops like corn could further influence food prices. Accurately assessing the environmental impact of feedstocks and production methods is also challenging due to differences in models such as the International Civil Aviation Organization (ICAO) Carbon Offsetting and Reduction Scheme for International Aviation (CORSIA) and Argonne Greenhouse gases, Regulated Emissions, and Energy use in Transportation (GREET).
Feedstock availability is a significant hurdle, especially for HEFA feedstocks such as waste oils, which are inherently limited. While agricultural feedstocks could theoretically be expanded, competition from sectors like renewable diesel poses challenges. Emerging PtL technology—utilizing abundant water and CO2—offers a solution. However, the early-stage and energy-intensive nature of the production process leads to high production costs. Moreover, its environmental benefits hinge on sustainable sourcing (e.g., renewable electricity for the electrolysis of water).
The financial landscape for SAF is challenging, with high production costs and market risks deterring private investment and making renewable diesel more appealing for producers. Government support and clear incentives are crucial for its economic viability. Additionally, the infrastructure for distribution and blending with conventional jet fuel requires further development. Finally, the low aromatic hydrocarbon content in SAF presents a dual aspect: it is an opportunity for reducing emissions, a significant environmental benefit, but simultaneously a challenge, as it can compromise seal integrity in older aircraft engines, necessitating new, compatible seal technologies.
In the longer term, hydrogen and electric aircraft may also help meet the aviation industry’s sustainability and environmental goals. However, both face distinct challenges. Most importantly, they have lower passenger capacity and range than conventional aircraft due to the lower energy density of alternative fuels compared to kerosene, though hydrogen aircraft have an edge over electric counterparts.
Using hydrogen, in combustion engines or fuel cells, significantly reduces carbon emissions and other air pollutants. For storage, aircraft rely on liquid hydrogen, which is space efficient but requires advanced cryogenic technology, or compressed hydrogen gas, which is less efficient and requires high-pressure systems. The International Council on Clean Transportation (ICCT) estimates indicate that by 2035, liquid hydrogen could enable narrow-body planes to carry 165 passengers over 3,400 km and turboprops 70 passengers over 1,400 km, making hydrogen-powered flights commercially feasible. In 2018, about one-third of aviation CO2 emissions originated from flights under 1500 km; ICCT’s projections suggest that hydrogen aircraft could potentially replace these emissions long-term.
Key challenges in their deployment include safety concerns, mixed public perception due to historical risks, and extensive infrastructure needs for hydrogen storage and handling. Refueling with passengers onboard may not be feasible, adding to airport operational costs. Producing and delivering hydrogen is also costly and complex, with higher costs for cleaner hydrogen. Moreover, ensuring environmental justice in deploying hydrogen solutions is essential to address disparities in the hydrogen value chain. Hydrogen aircraft could also increase contrail formation, impacting climate change. Finally, scaling up requires collaboration across various sectors.
Electric aircraft, on the other hand, have zero in-flight emissions and lower maintenance needs but are severely constrained by battery technology. This limitation affects their range and payload, confining them to very short flights. ICCT estimates indicate that even if battery technology improved to double its current efficiency, electric planes seating around 90 passengers may only cover distances up to 280 km. This limited range means they would have a minimal effect on aviation’s climate impact. However, they could still improve air quality by reducing the number of jet-fueled flight departures. Widespread adoption requires advanced charging infrastructure and potentially major power grid upgrades. Their environmental benefits also rely on whether the energy for charging them comes from renewable sources. Recent advancements are making short-range urban flights feasible, with studies suggesting electric vertical takeoff and landing aircraft could reduce emissions more effectively than electric cars for short distances.
Hybrid aircraft technologies, which utilize a combination of energy sources in flight are emerging as a promising solution. More developed than solely electric or hydrogen-powered alternatives, these technologies can be more readily adapted to fit current aircraft designs.
Voluntary changes in passenger behaviors: Another challenge is persuading passengers to make sustainable choices, such as rail, carbon offset programs, or flights with lower emissions. Many prefer air travel for its speed and convenience, even for short distances, despite the availability of greener alternatives. Encouraging a shift to rail for shorter trips involves improving its infrastructure, competitive pricing, and raising public awareness, which can be costly and complex. Carbon offsets provide an alternative, but their effectiveness is debatable due to validation challenges and potential community impacts. Moreover, getting passengers to choose lower-emission flights is complicated by inconsistent information from various flight comparison sites and the lack of a standardized emissions calculation method.
Operations and management: Efforts to improve aviation operations, such as optimizing flight schedules and engine settings, can further help reduce emissions but require a delicate balance between minimizing air quality impacts, cutting CO2 emissions, and maintaining passenger satisfaction. Research shows that reducing CO2 emissions can sometimes increase population exposure to other pollutants. Additionally, efforts to reduce CO2 emissions by flying slower can lead to longer flight times, potentially impacting passenger experience. Finally, decarbonizing airport ground operations, such as by electrifying ground support equipment and shuttle buses and improving the energy efficiency of airport buildings, can further help reduce this industry’s impact but requires comprehensive grid infrastructure upgrades, financial investment, technological innovation, sustainable energy sources, operational changes, training, and supportive policies.
Unclear national responsibilities: Under the United Nations Framework Convention on Climate Change (UNFCCC), emissions from international flights are recorded separately from individual countries’ national totals. Instead, they are classified under “bunker fuels” due to their occurrence in international waters or airspace, complicating efforts to hold specific nations accountable. During the development of the 1997 Kyoto Protocol, countries agreed to address emissions from these bunkers through the ICAO negotiations and not through the UNFCCC. Whether causal or not, this allowed countries listed as Annex I Parties to increase their emissions from these sectors without impacting their emission reduction commitments. For example, US aviation bunker fuel emissions doubled from 1990 to 2019.
Although the 2015 Paris Agreement encompasses all anthropogenic emissions, its lack of specificity has resulted in nationally determined contributions (NDCs) submitted by parties primarily focusing on domestic emissions, leaving international transport largely unaddressed.
CORSIA, introduced by ICAO in 2016, aims to manage emissions from international aviation, focusing mainly on airlines. However, effective emission reduction requires stakeholder involvement beyond just airlines, underscoring the need for countries to be responsible for these emissions.
Policy Solutions
Policies to promote the production and usage of SAF: Financial incentives, such as grants and tax credits, can reduce high production and usage costs, supporting feedstock cultivation, infrastructure, and research and development (R&D). Examples of such support include the US Department of Energy’s loan guarantees for commercial-scale SAF projects and the tax credits for SAF under the Inflation Reduction Act (IRA).
Market-based strategies, such as the European Union’s emissions trading system and ICAO’s CORSIA, can encourage airlines to use SAF. This approach can also indirectly promote developing more fuel-efficient aircraft by increasing demand.
Government investment in R&D can foster SAF innovation, efficiency, and scalability, making it more viable for the industry. A notable example is the governmentwide SAF Grand Challenge by the US Departments of Energy, Transportation, and Agriculture and other federal agencies launched in 2021 to help reduce costs and expand production and use of SAF.
Mandates that require blending SAF with conventional fuels can boost its attractiveness for airlines. An example is the EU SAF mandate approved in 2023 under the ReFuelEU Aviation initiative. Imposing taxes on fossil fuels can further incentivize the shift toward SAF.
Policies to advance aircraft technologies: Strict standards for aircraft emissions, such as those introduced by the ICAO for CO2 and nonvolatile particulate matter (nvPM) emissions, can encourage aircraft manufacturers to invest in R&D to meet or exceed these benchmarks. Concurrently, government investment in R&D, through initiatives such as the US Federai Aviation Administration’s (FAA) Continuous Lower Energy, Emissions and Noise and Eliminate Aviation Gasoline Lead Emissions Programs, can advance aircraft performance and reduce emissions.
Furthermore, government subsidies for R&D, demonstration, and deployment of emerging technologies, such as hydrogen and electric aircraft, can help bring these technologies to the market and improve their range and passenger capacity. They could be viable for short-haul travel and offer significant environmental benefits and reduced noise levels. Due to their early-stage development, they are likely to receive inadequate private investment, making government support essential. Yet, current US incentives for them lag behind those for ground vehicles, indicating a need for increased support in this sector.
Policies to change consumer behavior: Investing in high-speed rail can reduce air travel dependency for short-haul travel, provided it is competitively priced. Taxing air passengers, such as the UK Air Passenger Duty, can also encourage shifts to other transport modes. Furthermore, regulating the Voluntary Carbon Offset (VCO) market can help validate the authenticity and environmental benefits of carbon offset projects and aid buyers in comparing and understanding different carbon offset options. Finally, corporate policy changes, such as encouraging virtual meetings, opting for lower-emission transport, and limiting nonessential travel, can significantly reduce business travel’s environmental impact.
Policies to build sustainable airports: Financial incentives for renewable energy, electric ground service equipment, and hydrogen and electric aircraft infrastructure can encourage airports to embrace sustainable infrastructure and practices, reducing carbon and noise pollution and improving air quality. Over the longer term, policy reforms could streamline environmental reviews under the National Environmental Policy Act to accelerate airport electric and hydrogen aviation infrastructure development.
Policies to promote countries’ accountability: CORSIA and ICAO’s long-term global aspirational goal for international aviation are steps forward in managing international air travel’s environmental impact but do not assign emissions responsibility from international flights to countries. Including these emissions in the NDCs under the Paris Agreement is being discussed as a strategy for comprehensive accounting. The EU and its 27 member states already include emissions from outgoing flights in their NDCs. Environmental advocacy groups are pushing countries to revise their NDCs to cover all aviation emissions, although accurately attributing carbon emissions from international aviation to specific countries is a complex challenge.
Open Questions
Questions related to sustainable aviation technologies: Research can help determine the cost-effectiveness of modernizing versus retrofitting older aircraft and the optimal timing for fleet replacement, weighing the economic and environmental benefits of new aircraft against the impacts of production and disposal. It is also necessary to fully understand the effects of various emissions, including NOx, CO2, and particulates, the effectiveness of emission reduction technologies, how aircraft manufacturers respond to regulations (such as ICAO’s standards), and the true impact of policies on aircraft fuel efficiency.
Research is also essential in answering questions centered around SAF. It can help identify and resolve bottlenecks in maintaining a consistent feedstock supply. A key area is optimizing resource allocation, given the competition for feedstock and biorefinery capacity between renewable diesel and SAF. It is also crucial to understand the infrastructure and financing requirements for integrating SAF into aviation and analyze how international and national regulations, incentives, and mandates affect SAF pricing, adoption, production, and ticket prices. Research is needed to investigate the holistic environmental impacts, including ILUC, of various feedstock and pathways.
Research can also develop strategies for deploying and scaling hydrogen and electric aviation by helping improve production, storage, and transportation; assessing competition for hydrogen across sectors and strategies for efficient distribution; assessing environmental justice issues across hydrogen value chain; and exploring infrastructure needs, including flight patterns, refueling strategies, network design, and the viability of hub-and-spoke systems. It can also evaluate the life-cycle environmental effects of different technologies and compare the costs and benefits with alternatives such as high-speed rail. As regulatory bodies, such as the Federal Aviation Administration and European Aviation Safety Agency, develop guidelines on new technologies, research can play a crucial role in shaping them. Finally, research can help assess consumer attitudes toward new aviation technologies to steer their development.
Questions related to consumer behavior: Understanding consumer behavior is crucial for shaping sustainable transportation policies. Research can highlight how factors such as pricing, comfort, and demographics influence transport choices and willingness to pay for sustainable aviation. These insights are vital for devising strategies that encourage consumers’ shift toward greener aviation and alternative transportation. Research can also help assess the effects of policies such as aviation taxes and limits on short-haul flights on passenger behavior, ticket prices, and air travel demand; understand the role of loyalty programs in influencing flying frequency; and identify the impact of strategies, such as frequent-flyer taxes, on the travel habits of frequent flyers. Moreover, further research is needed to regulate VCOs to ensure they result in genuine, additional, and verifiable emission reductions.
Questions related to airport operations: The impact of airport operations on nearby populations, influenced by factors such as wind direction and flight frequency, is a key research area for mitigating adverse effects. Additionally, research can help optimize airport operations for environmental and passenger service improvements and develop ways to improve the setup of alternative technologies, such as hydrogen and electric aircraft systems.
Questions related to countries’ responsibilities: The effective attribution of carbon emissions to individual countries is an open question. Research and policy engagement can help develop a globally accepted method to distribute carbon-offsetting responsibilities.
Authors
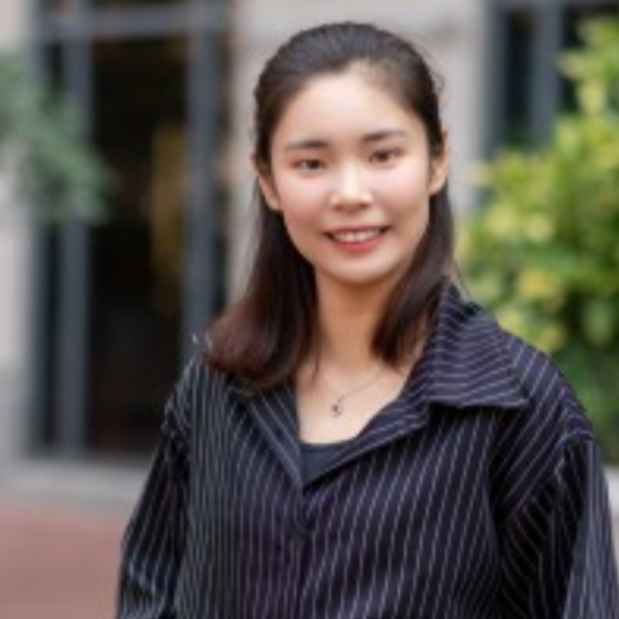
Zhiqing (Phoebe) Wen
Harvard University