Renewables 101: Integrating Renewable Energy Resources into the Grid
An exploration of how renewables connect to the grid, how these connections impact grid operations, and implications of a high penetration of renewables for the grid in the future.
Generating electricity using renewable energy resources (such as solar, wind, geothermal, and hydroelectric energy) rather than fossil fuels (coal, oil, and natural gas) reduces greenhouse gas emissions from the power sector and helps address climate change. While renewables are preferable to fossil fuel generators from an emissions standpoint, power output from renewable sources depends on variable natural resources, which makes these plants more difficult to control and presents challenges for grid operators.
To properly balance electricity supply and demand on the power grid, grid operators must have a sense of how much renewable energy is being generated at any given moment, how much renewable energy generation is expected, and how to respond to changing generation. All this information can be difficult for grid operators to know due to the intermittent nature of renewable power and the wide variety in the size and locations of renewable energy resources across the power grid. As the proportion of renewable energy capacity on the grid grows, these issues are becoming increasingly important to understand. This explainer explores how renewables connect to the grid, how these connections impact grid operations, and implications of a high penetration of renewables for the grid in the future.
This explainer frequently references the workings of the electric grid. To learn about how the grid functions and find definitions of some common terms, read “Electricity 101 :Terms and Definitions.”
How Is Renewable Energy Integrated into the Grid?
There are two main types of renewable energy generation resources: distributed generation, which refers to small-scale renewables on the distribution grid where electricity load is served; and centralized, utility-scale generation, which refers to larger projects that connect to the grid through transmission lines.
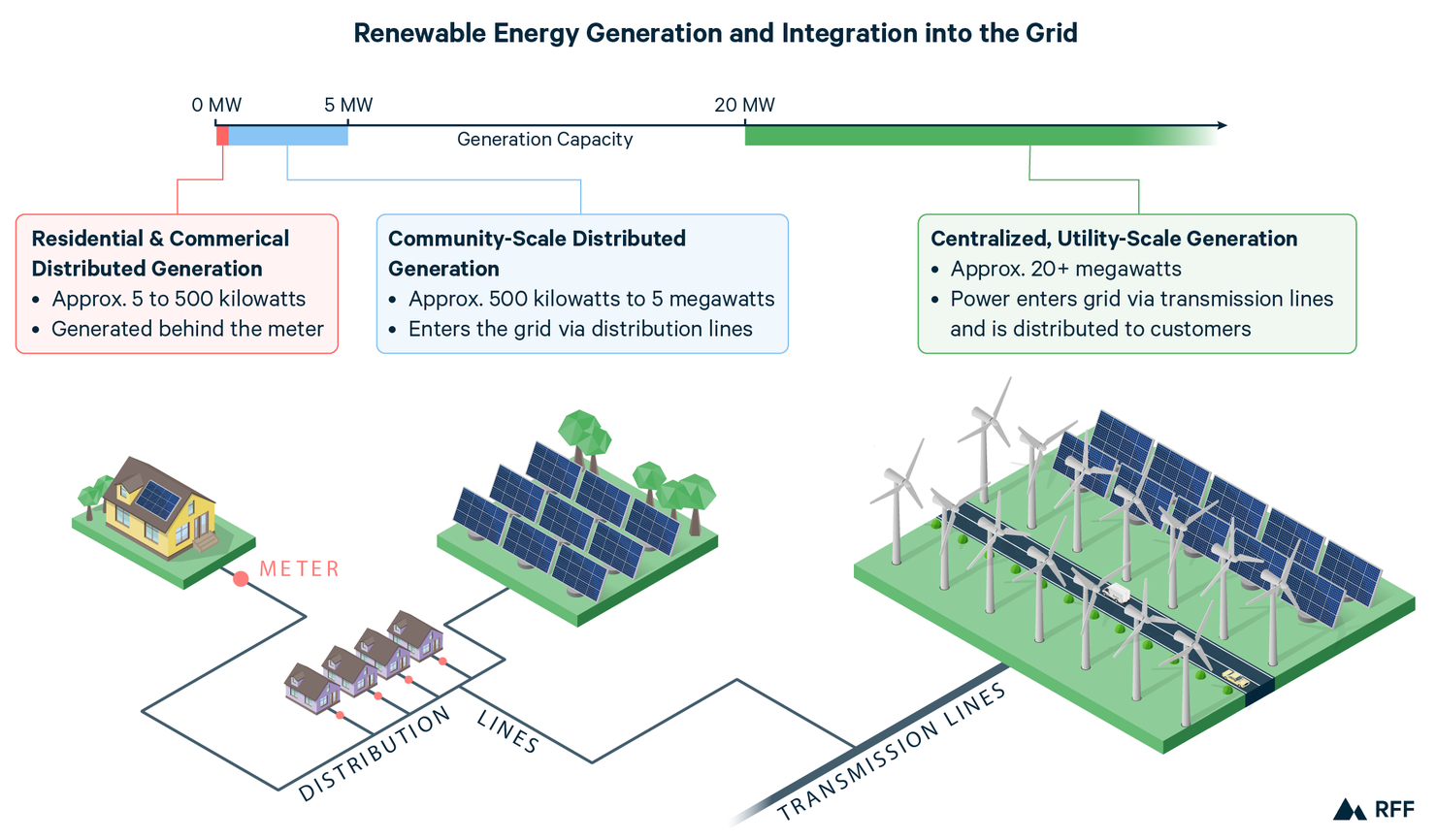
Utility-Scale Generation
Centralized, utility-scale renewable energy plants are comparable to fossil-fueled power plants and can generate hundreds of megawatts (MW) of power. Like natural gas, coal, and nuclear plants, large renewable plants produce power that is sent across transmission lines, converted to lower voltage, and transmitted across distribution lines to homes and commercial buildings.
Unlike conventional fossil-fuel plants, however, renewable energy plants are typically not dispatchable (or able to generate power when called upon), because they depend on variable resources like the sun and wind that change over the course of a day. However, when renewable energy is available, sources like wind and solar get priority in the dispatch order. Wind and solar have zero fuel costs, so their production is used before other generator types because they are the cheapest energy source available at that time. (To better understand how electricity generation is dispatched, read “Electricity Markets 101.”)
Distributed Generation
On the other end of the spectrum, small residential and commercial renewables typically range between 5 and 500 kilowatts (kW). Most of these small-scale renewables are solar panels, which are easily customizable in size (for a breakdown of solar types, see page 3 of this RMI document). These distributed resources, such as rooftop solar panels, are typically located on-site at homes or businesses. Unlike large, centralized renewable plants that connect to the grid through high-voltage transmission lines, distributed resources like these are connected to the grid through electrical lines on the lower voltage distribution network, which are the same lines that deliver electricity to customers.
Oftentimes, these projects occur “behind the meter,” which means that the electricity is generated for on-site use (such as a rooftop solar system that supplies a household with power). These small, distributed projects typically lower the demand for electricity at the source rather than increasing the supply of power on the grid. For example, when the sun is shining, a house that has solar panels on its roof may not need electricity from the grid because its solar panels are generating enough electricity to meet the residents’ needs.
Community-scale renewables, which are larger than rooftop projects but smaller than utility-scale, are also connected to the grid through distribution lines and are therefore also considered to be distributed generation. Unlike small rooftop renewables, however, community-scale renewables reside “in front of the meter,” meaning that the power they generate is not used on-site but rather flows onto the distribution grid to be used by homes and businesses in the vicinity.
Comparing Generation Types
Both centralized- and distributed-generation renewables have benefits and costs for customers and grid operators. From an economic perspective, centralized utility-scale renewables are much cheaper than distributed resources due to economies of scale. As of November 2018, the levelized cost (the net present value of the cost of electricity generation over a plant’s lifetime) of rooftop solar was estimated to be anywhere from 4.5 to 7 times more expensive per MWh relative to utility-scale solar.
In addition to being cheaper, centralized projects are often much easier for the grid operator to control. Because distributed renewables are often small and behind the meter, they can be very difficult to track from a grid operator’s perspective and can significantly complicate load forecasting. Grid operators usually only know that these projects exist because they noticeably reduce customer demand for electricity during certain times of day.
However, distributed renewables can provide the grid with benefits that large projects cannot. Since the energy from distributed generation is typically used on-site or nearby, distributed energy resources can significantly reduce energy losses that occur when electricity is carried on transmission lines, and they can avoid the cost of new transmission and distribution infrastructure (see NREL and Acadia Center). If they are connected to microgrids, distributed renewables can also provide greater resilience during storms that disrupt the power grid by providing power even if the larger grid experiences outages.
How Does Renewable Energy Affect the Grid?
Regardless of where renewable energy generation is located on the grid, it impacts how the grid operator dispatches resources in the same way. Most of the time, the grid will absorb all the electricity produced by renewables because there is sufficient demand for electricity. (During rare events, production from renewables exceeds demand for electricity in a given region and production must be curtailed. This may occur more often as the penetration, or proportion, of renewables on the grid rises. See here for more.) Consequently, grid operators only need to use other sources to make up the difference between the amount of electricity demanded and the amount of electricity produced by renewables on the grid. This is known as net load, which is equal to the difference between the forecasted load (the expected level of electricity demanded by customers), and the production of all renewables on the system. Utilities are responsible for meeting the net load and typically use conventional fossil-fuel resources, like natural gas plants, to do so. As a result, the more renewable energy resources present on the grid, the less electricity must be generated using conventional fossil-fuel plants.
However, as more renewables are integrated into the grid, their intermittent nature can pose problems for grid operators in terms of forecasting and meeting load. A growing proportion of renewables on the grid makes weather increasingly important for forecasting net load. Since weather can change quickly and unpredictably, high renewables penetration requires grid operators to be flexible and quickly react to new conditions and production patterns. Failure to do so could potentially lead to power shortages and blackouts.
Even if the weather is predictable, grid operators face the issue of how to quickly respond to dwindling production from solar energy when the sun goes down but the demand for electricity stays the same (or increases during late afternoon peak).
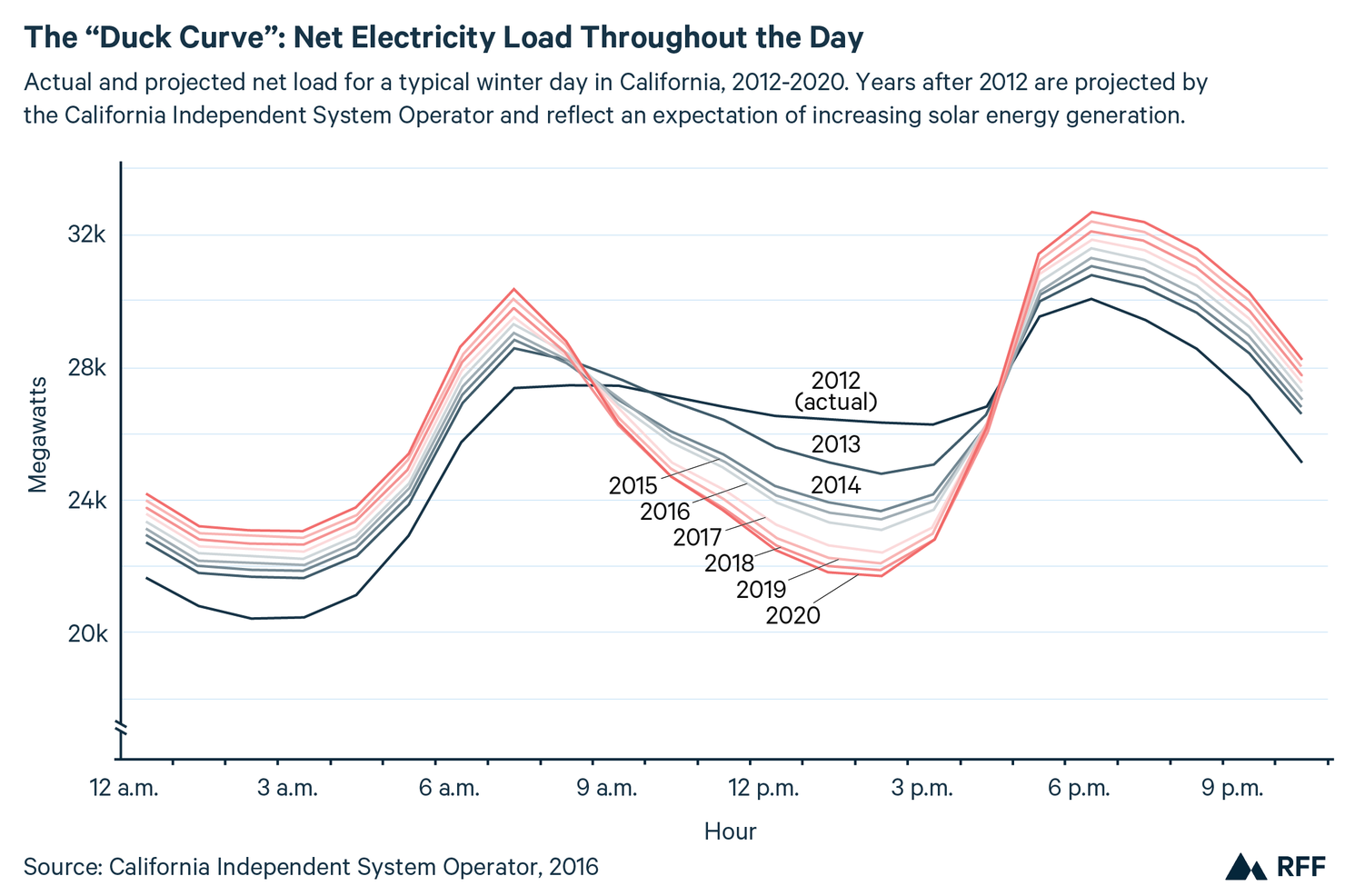
This net load curve is from the California Independent System Operator (CAISO), a system with a growing penetration of solar energy. As shown above, balancing grid operations in this system requires a very steep “ramp,” or rapid dispatch of non-renewable grid resources to meet electricity demand, in a very short period (between the hours of 4 and 8 pm) while the sun is setting to make up for the lost solar electricity production. This curve—often called the “duck curve” because the difference between the actual load and the net load looks like the body of a duck—illustrates the difficulties of balancing and controlling a system with a high penetration of renewables. The plot shows how, as more electricity comes from solar energy, the “belly” of the duck becomes deeper. A deeper belly requires more drastic ramping when the sun sets, which is often done using fossil fuel resources like natural gas. It also increases the risk of curtailment of solar energy. As mentioned above, grid operators will occasionally have to curtail, or reduce the output from, certain renewables during times of high production if there is not enough demand to utilize it, which can result in economic losses for renewable generators and a loss of clean energy production. Consequently, adding solar energy to the grid becomes less and less useful for both meeting electricity demand and reducing carbon emissions as the number of resources increases. This could be addressed by pairing production with energy storage to use later.
Implications for the Grid of the Future
Driven by falling costs and state and federal renewables policies, renewable energy is expected to grow significantly in the coming years. Some US states like New York, California, and Illinois have ambitious policies that require that most of their power must come from renewables within the next few decades. Hawaii, for example, plans to generate 100 percent of its electricity from renewable energy sources by 2045. To accommodate a high penetration of variable renewable energy, the modern grid will require a great deal of flexibility on both the electricity supply and demand sides.
There are several ways to increase grid flexibility and improve the integration of renewable resources:
- Energy storage can be paired with variable renewables to accommodate fluctuations in renewable generation over the course of the day or several days. Electricity can be stored during times of high generation (for solar, during sunny days; for wind, during times of high wind speeds) for later use during periods of high demand.
- Building more transmission lines to connect areas endowed with plentiful renewable resources (e.g., very sunny or windy areas) with areas of high electricity demand can increase the value of renewable resources and reduce uncertainties of their electricity generation. For example, building solar plants in deserts that get sunshine most of the year can reduce weather-dependent uncertainty associated with the system’s production.
- Combining different renewable sources can balance out variability of production of one resource type. For example, a grid that relies on many types of renewables—such as solar, wind, hydro, and geothermal all together—will likely face less volatility in production than one that relies exclusively on wind energy alone.
- Demand-side management can be used strategically to optimize the times when electricity is used to reflect the availability of generation. Demand-side management can include shifting flexible demand to times of high renewables production, such as preheating electric hot water heaters or charging electric cars during times of high solar energy production, and reducing demand during peak hours, thereby lessening the slope of the ramp.
- Placing value on generator flexibility in the wholesale power markets can encourage grid flexibility. California, for example, has attempted to manage its “duck curve” through the development of a flexible ramping product that compensates generators for their ability to ramp quickly. Options like this allow the market to decide how to best enable flexibility, rather than prescribing a set solution.
Renewables pave the way for a low carbon future, but the impacts of their intermittency on the existing grid system cannot be ignored. It will take advanced technology, careful planning, and increased flexibility to ensure a smooth transition to a renewables-dominated electrical grid.
For more information on improving flexibility and integrating renewables, please see this study by NREL.