Reforming Resource Adequacy Practices and Ensuring Reliability in the Clean Energy Transition
This report introduces the emerging challenges for resource adequacy planning, reform options, and insights from a February 2023 RFF workshop of subject matter experts.
1. Introduction
The Inflation Reduction Act and various state policies to encourage decarbonization of the power sector are expected to accelerate the proliferation of renewable energy. These resources, though critical to reducing carbon emissions, present some challenges for traditional resource adequacy frameworks, which are designed to ensure that sufficient energy is available to meet electricity demand and to prevent system blackouts. Intermittent resources and storage capabilities are often difficult to integrate into traditional frameworks because the reliability services they provide depend on the entire resource mix, the season, and even the time of day. Independent system operators and state regulators, who oversee resource adequacy in many jurisdictions, are considering a wide variety of reforms to more accurately evaluate how the diversifying energy mix affects reliability. Resource adequacy planners try to ensure that different resource types are properly compensated for their contributions to reliability and have appropriate incentives to perform when called. Approaches to reform could address factors that affect capacity demand, such as revisiting the basis for setting reserve requirements, and factors that affect supply, such as assigning capacity credit to intermittent and other resources that face uncertainties in fuel supply and extreme weather operation. This report offers an introduction to the emerging challenges for resource adequacy planning, options for reform, and insights from an RFF workshop of subject matter experts. The RFF event, hosted in February 2023, was subject to the Chatham House Rule, so specific experts cannot be referenced.
2. Resource Adequacy Today
Independent system operators (ISOs), regional transmission organizations (RTOs), and balancing authorities are responsible for ensuring that generation resources can meet electricity demand, The long-running industry standard is a once-in-10 years outage rate, though interpretations of this standard vary by region. subject to the long-standing industry standard of a once-in-10-year probability of an outage. Resource adequacy (RA) can typically be addressed through centralized procurement by a state or vertically integrated utility, an organized capacity market, or decentralized procurement by load-serving entities (LSEs) subject to common standards set by a central authority. Vertically integrated utilities that generate electricity, transmit to load centers, and act as load-serving entities also must plan for how to appropriately acquire enough resources to meet customer demand. All of these RA processes begin with planning and an assessment of future needs: an analysis of projected electricity load, including potential shocks to the system (such as an unexpected heat wave leading to increased energy demand for air conditioning), and consideration of a reserve margin that can accommodate these unexpected fluctuations in addition to changing energy supply. All RA planning methods analyze how the different resource types and generators in the system can be expected to perform under varying conditions.
The approaches to addressing shortfalls in supply revealed in these planning exercises differ across regions. In a decentralized RA procurement region like California, if shortfalls are predicted, then the state’s RA rules and guidance would prompt LSEs to go through a procurement process to purchase more generation capacity to close the gap. The California Public Utilities Commission (CPUC) establishes centralized guidance on the reliability value of different resources and how LSEs can calculate the reliability of their resource mix.
In contrast, a capacity market structure allows generators to bid independently to provide capacity. Capacity market operators like PJM require LSEs to purchase quantities of capacity that the operator has determined will be sufficient to meet peak demand plus a reserve margin. 15 percent is a common reserve margin in installed capacity terms, but some markets use lower UCAP targets. The system operators set up rules for which generators can participate in the market and what their capacity value is, based on their generation type and other factors. Generators can then make a bid (a specific quantity of capacity at a specific price) in the capacity market that reflects their willingness to provide capacity. Providing capacity obligates the resource to participate in energy and ancillary service markets, with potential exposure to penalties for failure to be available and/or deliver electricity when called on. Penalties are also applied in decentralized procurement approaches for LSEs that fail to adhere to resource adequacy requirements, and for suppliers that fail to meet capacity obligations.
Despite many differences among those RA approaches, the current challenges they face are similar: (1) new generation technologies complicate the task of estimating how much generation will be available at a given time; (2) methods of determining the RA contributions of different resources can have implications for the speed of grid decarbonization; and (3) overestimating electricity capacity needs can drive up costs for consumers, and underestimating needs can threaten reliability. These challenges call for novel approaches to RA planning, which is complicated by the risk-averse nature of ensuring reliability. Below we discuss areas of reform that are being explored across the United States.
3. Adapting Resource Adequacy Approaches for the Future
RA stewards must adapt to the evolving nature of electricity generation and demand as the grid decarbonizes. We explore five areas where RA planning and associated procurement mechanisms are changing: (1) how RA planners model future electricity demand and generation supply; (2) how considering the marginal value of additional resources can more efficiently identify needed resources; (3) how accrediting thermal resources similarly to renewable resources can help identify reliability gaps; (4) how penalty structures could be altered to provide incentives for performance in capacity markets; and (5) how calculating generator availability and demand by hour might more accurately capture system needs. We explore the motivations and challenges associated with each of these reforms below.
3.1. Modernizing Generation Modeling
RA modeling estimates future electricity demand and supply to determine the amount of generation resources needed to avoid shortages. Modeling analysis typically considers three interacting risk categories: unexpected surges in demand, systemic reductions in supply, and resource-specific reductions in supply.
Generally speaking, RA modeling is more accurate and useful if the modelers integrate more granular information about the risks associated with specific resources. Modelers seek detailed information about when generators of different types will be available, how generation equipment will perform under varying conditions, and how demand will fluctuate seasonally and even hourly.
However, even when detailed models are used and numerous future scenarios are considered, the data used to inform these analyses may not fully reflect future risk. Projections of supply and demand require assumptions that may introduce inaccuracies, but historical data may not predict future conditions. For example, what happened in Texas following the February 2021 storm Uri indicated that natural gas infrastructure could be unreliable in cold weather; however, infrastructure upgrades since 2021 may have reduced the likelihood of future shut-downs (TPUC 2022). Experts disagree about the extent to which the reforms to gas infrastructure in Texas fixed the issues that led to energy shortages during the 2021 event. The lack of consensus complicates the decisionmaking process for RA planners and informs the conversation in subsection 4. How do RA modelers incorporate new technology in their risk assessments, and how much should they rely on historical observations and data to predict the frequency of future outages? Climate change may further complicate this question if extreme weather events occur more frequently. Experts in the RFF workshop on resource adequacy highlighted the need to understand how low-frequency, high-impact events factor into resource adequacy planning, which should be modified to better capture future risks.
Changes in demand profiles also pose an opportunity for improving modeling. Increasing electrification could dramatically change peak loads or shift their timing (Bistline et al. 2021). Many models used for RA analysis depend on assumptions about how much flexible demand will be available to the system (CPUC 2019), but the extent of demand-response opportunities and how they could shape load curves are uncertain. Increasing distributed generation at the household (rooftop solar) or campus (microgrid) level may also widen the gulf between system load and net peak load, particularly as the same renewable generation types proliferate at the utility level. These changes in demand on the grid are evolving rapidly and are highly subject to federal and state policy changes. RA planners may need to rely more heavily on future projections of demand that deviate from historical trends.
3.2. Adopting Marginal ELCC
Adding new technologies to the generation mix can complicate evaluations of a resource’s contribution to reliability. Capacity markets allow resources with different capabilities to submit bids for their capacity services. The market operator then adjusts these quantity bids to create a fungible capacity product. Decentralized RA processes, such as in California, have also used resource adjustments to help LSEs identify the appropriate resource mix and the expected average contribution of each type of resource. Undervaluing resources can lead to over procurement; overvaluing resources can lead to shortages. Moreover, undervaluing or overvaluing some resources more than others distorts incentives, which can lead to an inefficient mix of capacity and generation. If offers by generators to sell into the capacity market accurately reflect their ability to meet system demand, bids can be accepted based on lowest cost per MW alone.
The most common way of adjusting resources’ capacity to reflect reliable capacity is known as effective load-carrying capability (ELCC). ELCC traditionally signals a resource’s ability to meet demand at times of peak load when resource adequacy is in question. Specifically, ELCC measures a resource’s contribution to reliability as the incremental demand for power that can be satisfied by the addition of that resource to the system (Garver 1966). ELCC is often expressed as a percentage of a resource’s “nameplate” capacity that reflects how much a resource can physically generate at a given time. Intermittent resources like solar have a lower ELCC rating because they may not be available when a reliability event occurs. As a result, intermittent resource offers into the capacity market are “downrated” so they can be compared equally with firm resources like gas and nuclear.
Although ELCC is a common metric, the way it is calculated varies by region. For example, PJM currently uses an “average ELCC” method, which values a resource category’s contributions to capacity based on the category’s average contribution across all levels of market penetration, including both additional and existing capacity. In contrast, “marginal ELCC” values a resource category’s contributions to resource adequacy based solely on the marginal effect of additional resources, which declines because of correlated availability risks. Research by Aagaard and Kleit (2023) finds that both marginal ELCC and average ELCC methods distort the market compared with a first-best capacity market system, but the distortions of marginal ELCC are easier to correct.
Choosing marginal or average ELCC accreditation greatly affects the value of resources in the capacity market. One study finds that storage resources in New England that had an ELCC of 43 percent under the average method would be valued at only 17 percent under the marginal method (Olson et al. 2021). Another study found that solar resources in PJM could be rated at 20 percent under an average ELCC method but only 1.3 percent under a marginal ELCC regime (PJM Market Monitor 2021). The theory behind marginal ELCC suggests that these low accreditations are an appropriate signal that additional storage and solar do not offer as much reliability value when the supply is already saturated with those resources.
NYISO recently adopted a marginal ELCC approach to provide more accurate price signals for additional capacity investments and to lower costs for consumers by selecting and compensating resources based on marginal capacity contributions (Cavicchi and Wu 2022). NYISO’s approach utilizes a system-wide installed reserve margin (IRM) and locational minimum installed capacity requirements, which are then translated into unforced capacity requirements to determine how much capacity needs to be procured. It then rates resources based on the marginal ELCC of their resource class and a resource-specific derating factor. Resources bid into the capacity auction based on their downrated capacity. Resources are selected by lowest price until the unforced capacity requirements are met, and selected resources are paid the clearing price multiplied by the accredited value of their resource.
The marginal ELCC approach involves several challenges: (1) calculating a resource’s marginal reliability value requires more data about the future resource mix and is less predictable than the more general resource accreditation typically used in the average ELCC approach; (2) it may lead to market clearing when the amount of accredited capacity purchased meets net peak load but is insufficient to meet gross peak load, should that become necessary Marginal ELCC is designed to identify the riskiest hours, which may not coincide with peak load. This is not inherently problematic but may be a concern for risk-averse RA planners. (Newell et al. 2022); and (3) it may heavily devalue a class of resources when the decline in marginal value is particularly steep because each MW provided in capacity is evaluated at the low marginal quantity.
At the RFF workshop, experts considered whether very low and uncertain remuneration for renewable resources in a marginal ELCC framework could lead to insufficient investment incentives for low-cost, low-emissions generation, leading to higher energy costs and higher emissions. These concerns become particularly acute as the share of renewable resources grows and energy prices trend toward zero in a growing number of hours, implying a greater reliance on capacity markets to cover the costs of new resources. In scenarios with high renewable penetration, the marginal capacity value of additional renewables depends greatly on the amount of storage resources on the grid. With near-zero energy prices and near-zero capacity values leading to low renumeration in capacity markets, additional resources may not be built unless they are coupled with storage, since the package could help decrease reliability risks.
One novel approach to address the final challenge, suggested by a team at Astrapé Consulting, is to use marginal ELCC to determine the quantities that generators bid but compensate capacity generators based on their average ELCC (Carden et al. 2022). Under this approach, resources’ marginal ELCC would determine their position in the supply stack relative to other types of generators and which generators clear in the capacity market, but compensation for the winning generators would be based on the quantity of capacity defined by the willing resources’ average ELCC. Advocates for this approach argue it would produce the proper price signals promoted by marginal ELCC advocates but still compensate generators for their contributions to system more broadly, not just on the margin (Carden et al. 2022). In the RFF workshop, critics of this approach pointed out that it could encourage bid-shading by suppliers, thereby reducing the efficiency of the procurement and the mix of generators selected.
Discussions at the RFF workshop highlighted concerns about the lack of transparency in ELCC accreditation, especially marginal ELCC. Determining the marginal ELCC involves several steps and assumptions, such as defining an appropriate “marginal unit” of capacity for the purposes of accreditation. The methodologies involve assumptions and complex processes that are not necessarily subjected to robust stakeholder engagement and debate and can often be opaque. Workshop participants said that relying on marginal ELCC can create challenges for businesses looking to establish a long-term view of income streams, which can change dramatically between capacity auctions. Although marginal ELCC may be the best tool for highlighting resources’ comparative values during times of system strain, it may reduce the role of the capacity market in creating stable long-term investment signals for generators. Many experts in the workshop were open to the idea that additional products may be needed to provide these signals if the capacity market leverages a marginal ELCC framework. In several states, including New York, renewable energy credits, tax credits, procurement mandates, and other mechanisms serve this purpose.
3.3. Differentiating Resource Value
RA planners balance expected errors in their projections of resource supply and electricity demand. Consider an oversimplified RA plan: a load profile is estimated to determine likely demand; a peak demand is identified; the RA planner ensures that deliverable generation resources can meet peak demand (accounting for estimates of likely forced outage rates); and a small buffer (a reserve margin) is added to account for unexpected generation outages or demand surges. In this simplified scenario, all resources are treated as equally able to deliver generation. RA planners know, however, that different resources contribute different reliability value to the system. Which resource-specific traits actually get represented in the ELCC, and which ones end up in the “buffer” that is absorbed by the whole system and helps determine the level of the IRM requirement?
Conversations about this distinction are currently playing out in PJM. Historically, firm thermal resources have bid into the PJM capacity market with only a small adjustment to their installed capacity based on forced outage rates that are assumed to be independent across different generators. Although these resources are not as variable as renewable resources, they still face correlated outage risks related to fuel supply and plant performance during extreme weather events. To the extent these correlated outage risks have actually been captured in RA planning, they have historically been reflected in the reserve margin at PJM (the total amount of capacity the ISO estimates is needed to ensure a reliable system). The reserve margin is the “buffer” designed to absorb risks of high demand or low supply that RA planners think may not be accounted for in the resource-specific ELCC calculation. Meanwhile, wind and solar resources have faced dramatic adjustments to their eligible capacity in the capacity market due to their intermittency and correlated outage risk (Dison et al. 2022).
PJM has proposed that a more fair and efficient system would appropriately attribute resource-specific risks to resource-specific ELCC adjustments (likely marginal ELCC) for all resources (Levitt 2022). This approach would theoretically improve the comparison among resources in the PJM capacity market, but the RFF workshop featured a robust conversation on the complicated nature of tying resource characteristics to an accreditation value. Traits need to have a clear and predictable relationship to availability across cases to affect the probabilistic models used to establish accreditation values. For example, a firm fuel contract for a natural gas plant may indicate better access to fuel during times of shortage, but in some events, like extreme cold, fuel use is diverted to home heating demand regardless of contract terms. A firm fuel contract therefore may not indicate actual firm fuel supply. If PJM moves forward with a more widespread application of ELCC for fossil plants in its capacity auction, it will have to decide what plant characteristics can be effectively included in the ELCC calculation. To include factors in accreditation, PJM will need appropriate data to predict the effect of specific factors on generator availability under varying conditions.
It is important to note that adjusting thermal ELCCs may not change the generating capacity mix in PJM if the marginal unit in the capacity market is still natural gas. The degree to which the generation mix in the capacity market changes as a result of RA reforms depends on the extent to which thermal resources are downrated and how the reserve margin is adjusted in response to incorporating resource-specific ELCCs. For example, if the adjustment to thermal resource accreditation is very small, it may not make thermal resources less valuable than similarly priced resources, meaning the bid order in the capacity market would not change. Additionally, if the reserve margin is not adjusted proportionally to reflect risks newly reflected in resource accreditation, more resources, including more thermal resources, may be needed to meet the reserve margin. Regardless of the effect on generation mix and costs, RA approaches should accurately reflect each resource’s contribution to reliability.
3.4. Changing Penalty Structures and Incentives
After Winter Storm Elliot, PJM imposed $1 billion to $2 billion in penalties on multiple generators that failed to meet their capacity obligations (Howland 2023). Some were gas plants that were unable to access fuel, and some were solar plants that could not deliver because of cloud cover. Proceeds from these penalties will be distributed to generators that exceeded their obligations during the reliability event.
Such performance failures raise questions about the effective design of penalty structures. For example, if a solar resource is modeled as unavailable in the evening for RA planning purposes and its ELCC derated accordingly, should it still face penalties when it is called to perform in the evening? Penalties for nonperformance are an important accountability measure to give generators incentive to improve their availability, but are they effective when they punish generators for characteristics they cannot change without significant new investment (like adding storage to a solar facility)?
Penalties and accreditation methods may have some overlap that needs to be considered. In PJM, clean energy advocates have suggested that penalty structures be altered to reflect the assumptions made in the ELCC accounting (NRDC et al. 2022). For example, an intermittent resource generator without onsite storage would have a lower ELCC than its competitor with storage, but it would not be punished for failing to perform when the wind is not blowing. However, if it has an unplanned outage and cannot deliver when the wind is blowing, it would face penalties. This adjustment may lead to fewer penalties for resources with low ELCC valuations while also maintaining incentives for generators to improve characteristics that are not explicitly factored into the ELCC. Such a penalty structure would still provide an incentive for wind generators to add onsite storage to increase their ELCC or make improvements that would reduce outages to avoid penalties.
In the RFF workshop, critics of differential penalty structures identified the need to establish different products for these purposes, so that purchased units of capacity in a market are fungible. For example, a Brattle report recommended implementing a three-year forward clean energy market (FCEM) that would precede the capacity market (Spees et al. 2019) and could replace established renewable energy credit (REC) programs. An FCEM would allow LSEs and other energy buyers to submit demand for clean energy in the forward period and would clear lowest-cost resources. Having these two markets proximate to each other (or potentially co-optimized) would lead to more opportunities for clean generators to earn revenue and could limit pressure on intermittent generators to take on penalty exposure in the capacity market. Arguments against a differentiated penalty structure also highlight the simplicity of uniform penalties for providing market signals of reliability.
Penalties play an important role in ensuring the performance that generators are compensated for in a capacity market. Penalties may provide an important backstop for generators to conduct their own risk assessment of what capacity they can reliably provide. Participants in the RFF workshop discussed the difficulties of designing an adequate penalty system based on the frequency and severity of penalty events. If penalties are set too low, generators may be inclined to overpromise what they can deliver to make more money upfront in the capacity market, knowing only a fraction of their profits will be sacrificed to penalty payments. If penalty rates are set too high, risk-averse generators that could offer reliability value may not offer into the capacity market at all, driving up prices of capacity. The frequency of penalty events further complicates this balance. If penalty events happen very infrequently, firms may not be able to assess whether they are likely to face the penalty at all. For example, if penalties are extremely high but reliability events (triggering penalties) happen only once every 10 years, a generator may create a business model built on making money in the capacity market for as long as possible and then declaring bankruptcy when those high penalties are imposed. To address this issue, participants in the RFF workshop expressed interest in increasing the number of performance evaluation events where penalties could be levied.
Other compensation structures could help address performance and penalty issues. The PJM market monitor has suggested paying generators per MWh of observed availability during the compliance period, rather than compensating generators upfront based on their predicted availability, and then penalizing them only for being unavailable during capacity assessment events (PJM Market Monitor 2023). This would reduce the upfront payments to generators and could create greater incentives to meet performance expectations at all times. Participants in the RFF workshop also discussed allowing generators to bid with a specific load profile that accounts for their best expectations of when they will be available, with penalties based on deviations from that offered profile in real time. This design would help generators avoid the risk associated with bidding into the capacity market with full availability obligations at infeasible times and could give planners a clearer picture of what capacity generators expect to provide. Wolak (2021) proposes a different approach, whereby different types of resources can use contracts to bid jointly to supply different load shapes that LSEs are seeking to procure on a forward basis.
3.5. Considering Slice-of-Day and Seasonal Markets
As the resource mix diversifies, RA planners consider the characteristics of each resource and how it contributes to reliability. Renewables like wind and solar introduce a challenge because of their high variability over the course of the day and across days and seasons. This variability is sometimes predictable: there will be less solar power in the winter than in the summer, for example, and more wind at night than in the daytime. Some jurisdictions account for this predictable variability with an adjustment to the resource’s representative capacity value (see subsections 2 and 3). California is adopting a new approach that balances temporally granular information about when these resources are typically available with the transparency needed in a decentralized RA procurement process.
Since 2018, California, which operates a decentralized reliability procurement process subject to regulatory requirements, has used an ELCC approach to value resources in RA planning. This approach is increasingly difficult to implement with the proliferation of LSEs, each of which must independently fulfill RA requirements and keep up with rapidly changing ELCC calculations. The growth in community choice aggregators and electricity cooperatives has increased the number of entities serving load. In 2025, accordingly, the state will transition to a “slice of day” RA framework. The new approach alters the historically used peak-hour approach to a 24-hour profile that identifies generation gaps and surplus at different times of day (CPUC 2022). It is meant to provide clearer long-term signals by more accurately identifying opportunities for storage resources to contribute to resource adequacy and remove the existing caps (defined below) on certain resource types—a relatively blunt method for dealing with resource variability.
The caps, a constraint on how much an LSE can rely on resources with different energy and duration limits to meet its capacity needs, limit the LSE’s exposure to shortages in one type of resource (particularly storage resources), but they do not efficiently identify when those resources stop adding capacity value to the system. The caps were defined as a percentage of capacity that could be served by different resource types. Details can be found here: https://www.cpuc.ca.gov/-/media/cpuc-website/divisions/energy-division/documents/resource-adequacy-homepage/resource-adequacy-compliance-materials/resource-adequacy-history/8-23-2022-planning-reserve-margin/workshop-5_ed_220823.pdf For example, additional battery storage in the system might mean that additional solar capacity could be used to charge those batteries. Figure 1 shows an example day when surplus energy generation in the daytime slices feeds storage capacity that is then available to deliver power in the evening slices. Acquiring additional storage resources may create value for additional solar resources even when existing solar generation exceeds demand. In other words, the resource mix that can meet demand in each slice depends somewhat on the resources available in other slices. Participants in the RFF workshop likened this to a highly simplified marginal ELCC structure.
Figure 1. CA Slice of Day Example (September 2024)
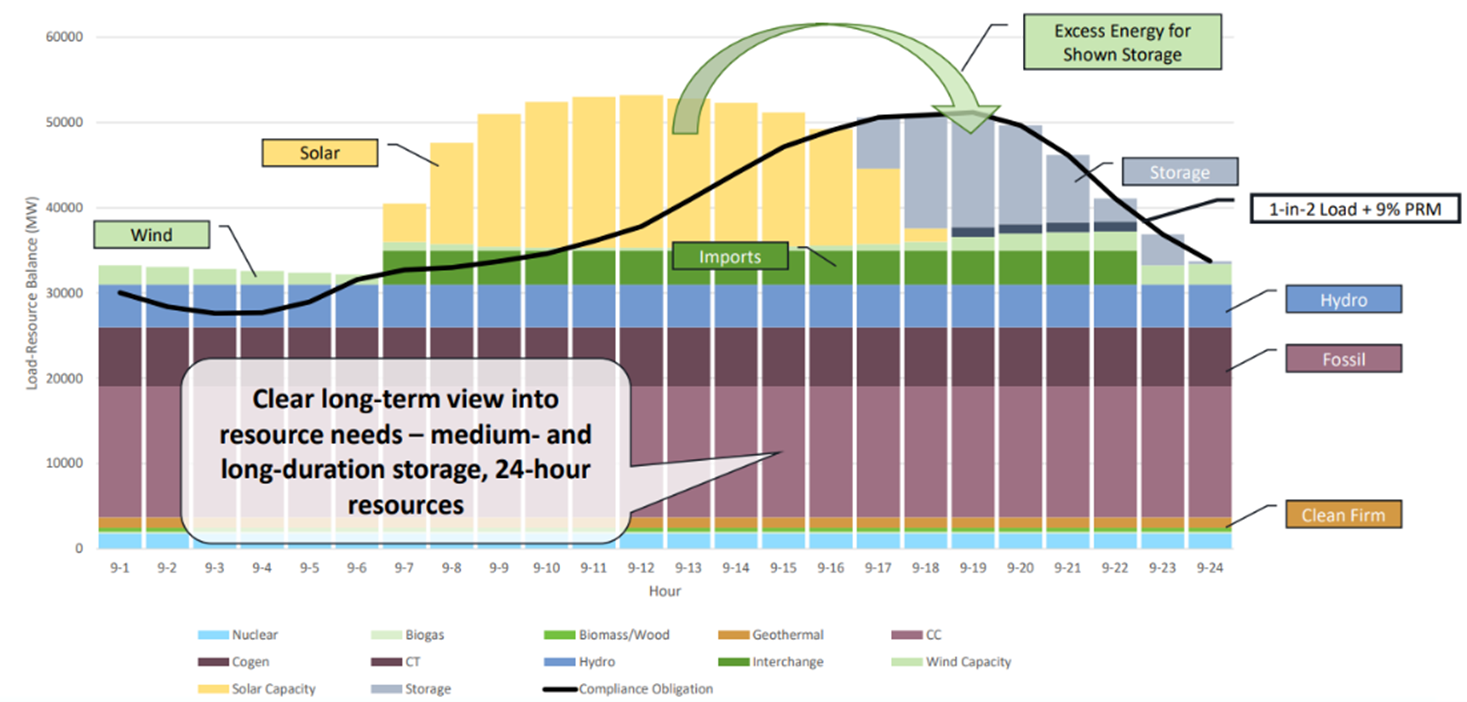
In the RFF workshop, experts discussed the factors driving adoption of the slice-of-day approach in California and the many remaining challenges. As a state where RA procurement is delegated to LSEs, California has prioritized a planning approach that is more transparent than ELCC approaches. Whereas ELCC values can change regularly (and sometimes unpredictably, from the perspective of LSEs), the slice-of-day model allows LSEs to directly observe how different resources interact to serve load. However, the simplicity offered by the slice-of-day approach means sacrificing some detail about resource availability included in ELCC modeling, such as correlated resource availability over the course of multiple days. RFF workshop participants expressed concerns about the investment signals provided by the simplified planning framework, but California experts stressed that the RA planning scheme is neither the only nor the primary driver of investment for new generation in the state. The integrated resource planning process, led by the CPUC, directs investments in generation across the state to meet long-term resource adequacy and environmental goals. Understanding the role of compensation associated with RA services for driving investment is essential to evaluating whether RA policies are effective and reasonable.
California’s approach to resource adequacy is in part a result of its decentralized process. Experts in the RFF workshop observed that this approach would not be needed if a centralized procurement option, like an ISO-run capacity market, were available. However, the California situation reveals how using ELCC frameworks may not be feasible in all jurisdictions, and how RA planners may need more transparent ways of representing RA needs as intermittent resources expand.
Taking a different approach to adding time-varying detail, both ISO-NE and PJM are looking at establishing separate seasonal capacity markets that consider the different risks to resource availability and different timing of potential spikes in demand during summer and winter temperature extremes. Figure 2 shows the projected daily net load profiles in PJM in summer and winter under different levels of electrification (“accelerated” refers to accelerated decarbonization of power generation and “accelerated with electrification” adds greater electricity demand). With growing electrification of heating technologies, more of the load-loss potential shifts to winter. The different shapes across the day and different timing of peak net load indicate a potential benefit of having two capacity markets, one for summer and one for winter. Specifically, aligning seasonal load expectations with seasonal resource availability can reveal different types of system strain that can be served by different resources. Proponents argue that seasonal markets, similar to the slice-of-day proposal, help lower costs by efficiently identifying resources that contribute to reliability on a more granular time scale (Graf 2022). A seasonal market may be more realistic than a slice-of-day approach in a region with a capacity market because hosting an auction for each slice of day for each month could be administratively burdensome. NYISO has a seasonally differentiated capacity market, and MISO has proposed a similar approach.
Figure 2. PJM Assessment of Net Load Risk under Energy Transition Scenarios (2035)
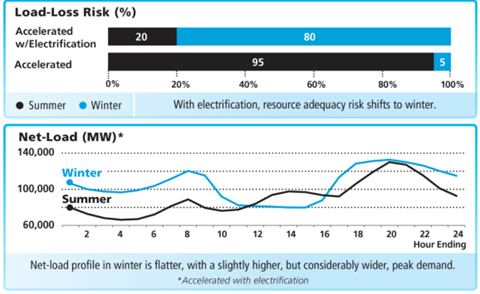
4. Conclusion and Remaining Questions
Different regions are taking a range of approaches to evolving their RA planning and compliance frameworks. With the ongoing proliferation of renewable energy, likely to be stimulated further by the Inflation Reduction Act, states, utilities, ISOs, and RTOs must consider how emerging technologies and a changing resource mix can both improve and challenge system reliability.
The RFF workshop that explored questions about resource adequacy mechanisms revealed several new directions for research. The ideas discussed fall into two categories: informing policymakers in the near term as they update their RA strategies, and reimagining how resource adequacy can best meet the system needs of the future.
Experts agreed that more research is needed to help inform ongoing policy decisions. For example, would introducing seasonal capacity markets in ISO New England improve reliability and lower costs by allowing for (1) a more specific accreditation process that captures seasonal variations in availability and (2) a more specific demand profile that reflects the expected timing of seasonal gross and net peaks? What role can demand response play in RA frameworks, and under what conditions can it really act as a replacement for generation? Given the dynamics and uncertainty in marginal ELCC values for new renewable resources, can marginal ELCC frameworks support investment decisions for renewable generators, or are additional products and revenue streams needed to promote investment? How should different levels of fuel assurances (firm fuel delivery contracts, on-site fuel storage, other) affect ELCC values for fossil generators? Understanding how these reforms influence reliability, costs, and generation mix is critical to building efficient and effective policy.
A more fundamental issue is how reliability will be treated and preserved in the future. Questions focus on accreditation, expanding RA products, and defining the optimal level of outage risk to inform system planning and reliability requirements. With respect to accreditation, participants asked whether generators, within an appropriately incentivized structure, could ultimately be allowed to create their own predicted generation profiles to sell—that is, defining their own quantities and periods of compliance when they believe they are likely to be available. This approach may reduce reliance on centralized accreditation methodologies to determine whether the resource mix is adequate to meet load needs, but it would likely require a greater role for performance penalties or other mechanisms to encourage truthful revelations and better performance when called. A fundamental question here is how to design mechanisms and approaches that help mitigate risk to investors but at the same time maintain incentives to deliver energy the moment it is needed and to conserve (or consume) electricity when it is expensive (or cheap). Another question is how such a system would be implemented in a capacity market framework versus a decentralized procurement process driven by LSEs.
Workshop participants also considered how many independent electricity products or new ancillary services (such as fast ramping or flexible capacity) should be created to meet system needs. Already, CAISO, MISO, and SPP have created a short-term ramping product to help accommodate loss of solar generation at the end of the day. What additional products might be required to balance load in the future, and how well are the new products delivering on expectations? Are there new products (such as rewards for state of charge) that would help make better use of energy storage products? Proliferation of products creates market complexity and may increase costs, but it may be better than relying on a more limited set of products that don’t fully address the wide range of system needs.
Finally, participants asked more broadly what level of risk of outages system operators and electricity regulators should be targeting in their reliability standards and requirements, and how the cost of meeting that risk target relates to what research tells us about the “value of lost load.” Although our understanding of the value of lost load and the factors that affect it is still evolving (Gorman 2022), there is a gulf between, on the one hand, academic research on optimal outcomes and consumer willingness to pay for reliable electricity and, on the other hand, the strong responses of energy consumers, their elected representatives, and appointed government officials when outages occur. Resource adequacy stewards often sit between these two opposing perspectives and struggle to find the appropriate balance.
Our work does not provide a comprehensive list of reforms being considered, and some experts are focused on even more dramatic solutions to improving planning for future reliability, including reforming energy markets to include an organized market for long-term contracts to encourage investment Four proposals for centralized long-term market designs were analyzed at an event cohosted by RFF and World Resources Institute in 2020, insights from which are available at https://www.rff.org/events/workshops/market-design-for-the-clean-energy-transition-advancing-long-term-approaches/ and summarized by Hausker and Palmer (2021). A standardized fixed-price forward contract has also been proposed (Wolak 2021). and relying more on interregional transmission to support greater connectivity between energy sources and loads. We will continue to explore these reforms and highlight their strengths and weaknesses in ensuring an efficient, reliable grid.