Forest Bioenergy 101: Generation and Emissions
Forest bioenergy has been heralded by some as a promising renewable energy source and condemned by others as having negative effects on the environment. Here's a review of the basics of forest bioenergy generation and emissions.
Forest bioenergy describes the energy generated from the combustion of wood and wood wastes or biofuels derived from wood. Woody material can be sourced from harvested trees or from forest biomass that would otherwise have been treated as waste—residues from a harvest, production of other wood products, or from urban waste streams. Forest bioenergy has been heralded by some as a promising renewable energy source and condemned by others as having negative effects on the environment. Most International Panel on Climate Change (IPCC) climate scenarios anticipate that bioenergy will play a critical role in reducing emissions from the energy sector. This explainer reviews the basics of forest bioenergy, the different ways of looking at its impact on the environment, and the different methods of measuring and counting forest bioenergy emissions.
How Is Forest Bioenergy Generated?
Forest biomass has long been used to generate thermal energy through the burning of firewood and residual material from wood product production. In the early 1800s, wood-based charcoal was the dominant commercial fuel source in the United States. Modern use of forest biomass increasingly involves compressing biomass to produce wood pellets (as compressed wood typically burns cleaner than uncompressed wood and is less costly to transport). The wood pellets are then burned to create electricity (typically co-fired with coal) or to generate heat. Any tree species can be used for bioenergy, but in the United States, wood pellet production increasingly uses fast-growing southern pine species (especially loblolly and slash pines).
Where Is Forest Bioenergy Used?
According to the US Energy Information Administration, in the United States, biomass electric generators are found in 40 states and generated 2 percent of total US electricity generation in 2018: solid wood materials represented 30 percent of that electricity output; black liquor, a byproduct of wood pulp production, accounted for 28 percent. As of 2019, most forest bioenergy harvesting occurred in privately-owned working forests, with about 75 percent of production in the Southeast and 17 percent in the Northeast. US forests are managed for the production of wood products as well as for resource benefits, such as wildlife habitat, water quality, or recreation. Management activities ( e.g., thinnings, prescribed burning, final harvesting, and passive holding) produce forest structures best suited for providing desired benefits. Management approaches vary, from intensive approaches with clearcutting and tree planting, to partial harvesting and natural regeneration, to no active management. Approaches also differ among ownership groups (families versus industry), forest types (pines versus hardwoods), and regions.
Europe consumes much more bioenergy than the United States, as both the European Union (EU) and the United Kingdom (UK) classify forest bioenergy as renewable as long as it meets certain standards (find the EU’s sustainability qualifications here and the UK’s standards here). The wood used to fulfill Europe’s demand for bioenergy mainly comes from wood pellets produced in the Southeastern United States.
Understanding Forest Bioenergy Greenhouse Gas Emissions
Using forest biomass for energy alters both carbon emissions and carbon uptake by forests. Depending on the approach to measuring these changes in carbon flows and the time frame under consideration, forest bioenergy has been characterized by some as a low-carbon energy source, carbon-neutral, or even carbon-negative; others claim that forest bioenergy adds net emissions into the atmosphere. There are several factors to consider when determining the emissions impacts of forest bioenergy, including whether a "closed carbon cycle" exists, the short-term and long-term effects of forest carbon uptake, and the choices made regarding land use and forest management.
Closed Carbon Cycle
The claim that forest bioenergy can be carbon neutral or carbon negative starts with the concept of a closed carbon cycle. This concept holds that a set amount of carbon dioxide cycles between forests and the atmosphere through natural processes. Forests absorb carbon dioxide through photosynthesis and accumulate carbon in plant biomass and forest soil. When trees and other biomass are harvested and burned to generate electricity, carbon is released back into the atmosphere. If the forest regrows, that carbon is reabsorbed. As long as new trees replace the ones harvested for energy, forest bioenergy releases no additional carbon from forests (though additional emissions from fossil fuels are released during the collection, transportation, and processing of biomass).
Short- and Long-Term Effects
Because trees are long-lived organisms, carbon emitted from today’s bioenergy generation will be reaccumulated over time as new trees grow on harvested forestland. As a result, forests that are harvested and regrown will cycle between emission and capture of atmospheric carbon dioxide. Across the managed forest landscape, forests are in all stages of development between harvesting and maturity. Without accounting for market effects that would increase forested area and the productivity of forests, expansion of forest biomass production would initially increase emissions as carbon uptake is spread across future years. The amount of time between the emission and recapture of carbon varies depending on the growth rate of different tree species and forest regions. Some research focuses on understanding this set of timing issues.
Land Use Changes
The notion of a closed carbon cycle depends on the retention of forestland. Changes in land use “open up” the closed carbon cycle: they imply either the loss of a carbon sink when forests are permanently replaced by another land use or new sinks when other uses switch to forest cover. Forest losses might occur if forest bioenergy were transitory—where the harvest serves as land clearing and land is converted to agriculture, developed, or another use. However, transition to forest bioenergy systems would increase wood demands and enhance net returns to forest ownership and management, which would encourage forest retention and afforestation. Strong growth in forest bioenergy markets might also raise timber prices and draw some land out of agricultural land uses and into forest biomass plantations or encourage afforestation of degraded lands (e.g., strip mine benches), generating a gain in the forest carbon sink.
Forest Management Changes
In addition to land use changes, forest management choices are influenced by returns to forest bioenergy. Especially in the areas with active bioenergy markets, management inputs (thinnings, fertilizer, etc.) would likely increase in response to prices, increasing rates of forest growth and carbon sequestration. For example, forest thinnings can produce more frequent woody biomass harvests and increase net biomass and carbon accumulation over the life of a managed forest. So, in the initial phases of a switch to bioenergy, existing forests could begin to accumulate more carbon than emitted—expanding carbon sequestration beyond amounts in a closed carbon cycle.
Predicting Net Emissions
Contradictions in the Literature
In order to design effective climate policy, decisionmakers need to have a clear understanding of how the substitution of forest bioenergy for fossil fuels could affect climate change. Studies of the greenhouse gas impacts of forest bioenergy have produced varying results and conclusions, in part because they have used different methodologies or failed to address certain factors. As outlined above, several interrelated biological and market dynamics ultimately affect net emissions from forest bioenergy. Results depend on the scope of the analysis, the time period considered, and the baseline to which bioenergy scenarios are compared.
A complete evaluation of biomass emissions includes elements from several fields, including biology (for forest growth and carbon uptake), engineering (for energy conversion efficiency and lifecycle effects), economics (for market dynamics and land use change), and forestry (for management possibilities and responses). Excluding any element yields an incomplete evaluation of effects and distorts the policy discussion. Partial analyses are not uncommon in the scientific literature and explain much of the disparities in findings across studies.
Can Forest Bioenergy Be Carbon Neutral?
Using a wood feedstock to generate energy has been equated with carbon neutrality, where carbon released from combustion is reabsorbed through regrowth, yielding no net increase in atmospheric carbon. With multiple effects on carbon flows, including market responses such as land use changes, it is unlikely that forest bioenergy is precisely carbon neutral. Rather, the key issue is a full accounting of carbon emissions associated with forest bioenergy at relevant scales. The result could be net zero, net positive, or net negative when compared to the use of fossil fuels. Studies that account for market effects over the long run indicate strong net benefits from using bioenergy.
The Role of Biological Single-Stand Models
The foundation of emissions accounting is a careful analysis of the emissions from burning various forest feedstocks and the uptake of atmospheric carbon through forest growth over time. The most basic form of this analysis is a model that describes the growth of biomass and accumulation of carbon over time of a single forest stand: a single-stand model. This type of analysis defines the amount of time required for emitted carbon to be recaptured by forest growth on a fixed area of land (repaying the “carbon debt” or defining the “payback period”).
Single-stand models provide useful measures of the relative emissions and forest recovery times across different tree species and broad regions, but they provide limited insight into policy futures because they are restricted to a fixed area of land and do not account for market-driven responses that also alter forest carbon stocks through changes in land use and forest management. Their harvest emissions and biological growth analysis can, however, be applied to more complete forest sector modeling efforts (see below).
Emissions and Life Cycle Effects
As with all sources of energy, bioenergy has emissions effects throughout its production process that need to be included in emissions calculations. Harvesting trees, transporting timber, and manufacturing products involve the use of fossil fuels and contribute additional carbon emissions. An accurate comparison of forest bioenergy emissions with fossil fuel alternatives requires accounting for emissions across their entire production processes.
Accounting for Market Effects
Forests in the United States are managed for several forest products, and a single forest may generate material for more than one market. Policies that expand demand for forest bioenergy feedstocks would influence the prices and outputs of these interrelated products, increase the returns to forest management, and expand demand for forestland. Going beyond a single-stand model to account for the sector as a whole requires addressing these land use, management, and product substitution effects. Forest sector models link market conditions to forest growth to predict the amount of production over time and changes in forest inventories and forest carbon dynamics.
“Studies that assume there is little to no management response, or consider only use of the extensive margin, predict that bioenergy demand will increase carbon emissions. Studies that allow efficient investments in forestry management find that bioenergy policies lead to a net increase in carbon sequestration.
A Case Study
A simple case study can illustrate the net emissions patterns of switching from fossil fuels to bioenergy.
A closed carbon cycle case study examines a simple hypothetical model of carbon flows for a case where forest feedstocks come from harvested trees and no market effects are considered. The chart in Figure A shows carbon dioxide recaptured by a forest harvested at year 5 with a 30-year regrowth period—a simplified single-stand model. The chart in Figure B shows how atmospheric carbon would accumulate for a fossil fuel source (contributing 1 gigaton of CO₂ per year) and for an alternative forest bioenergy source (contributing 1.1 gigaton of CO₂ per year) for the 30-year case as well as for a 60-year regrowth case. Each year, a harvest occurs and forest regrowth is initiated (consistent with Figure A). For fossil fuels, the amount of atmospheric CO₂ steadily accumulates over time; for forest bioenergy, it reaches a plateau at the end of the regrowth period. (The plateau is roughly 50 percent of the carbon released over the regrowth period at approximately 16 gigatons for the 30-year case; 32 gigatons for the 60-year case). This steady state represents the carbon flows between the atmosphere and living biomass at any given moment. As a result, switching to forest bioenergy results in net emissions reductions that increase over time (Figure C). For the 30-year case, resulting greenhouse gas concentrations are halved by year 31 (year 65 for the 60-year case) and are 84 percent less at year 100 (68 percent less for the 60-year case).
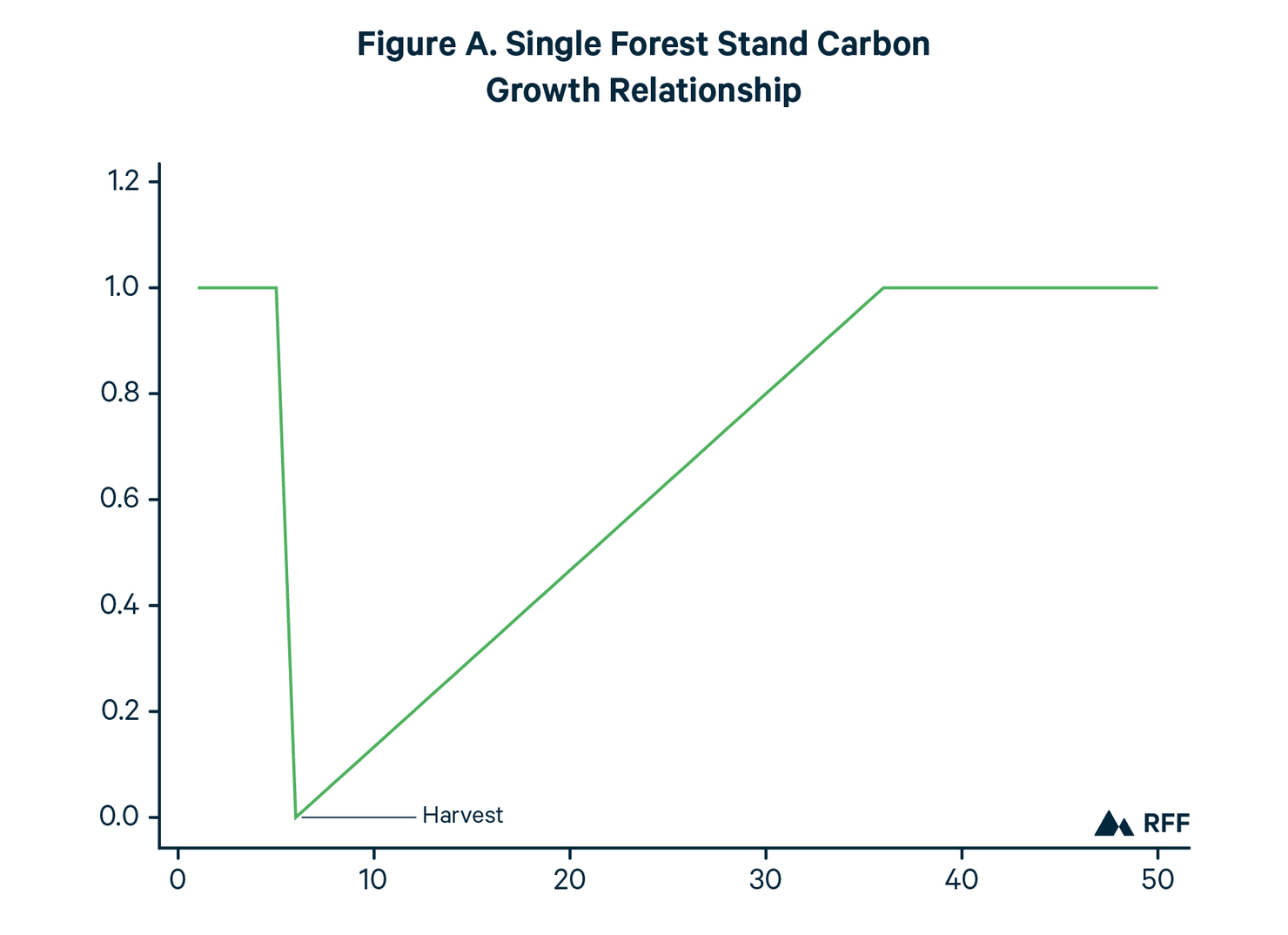
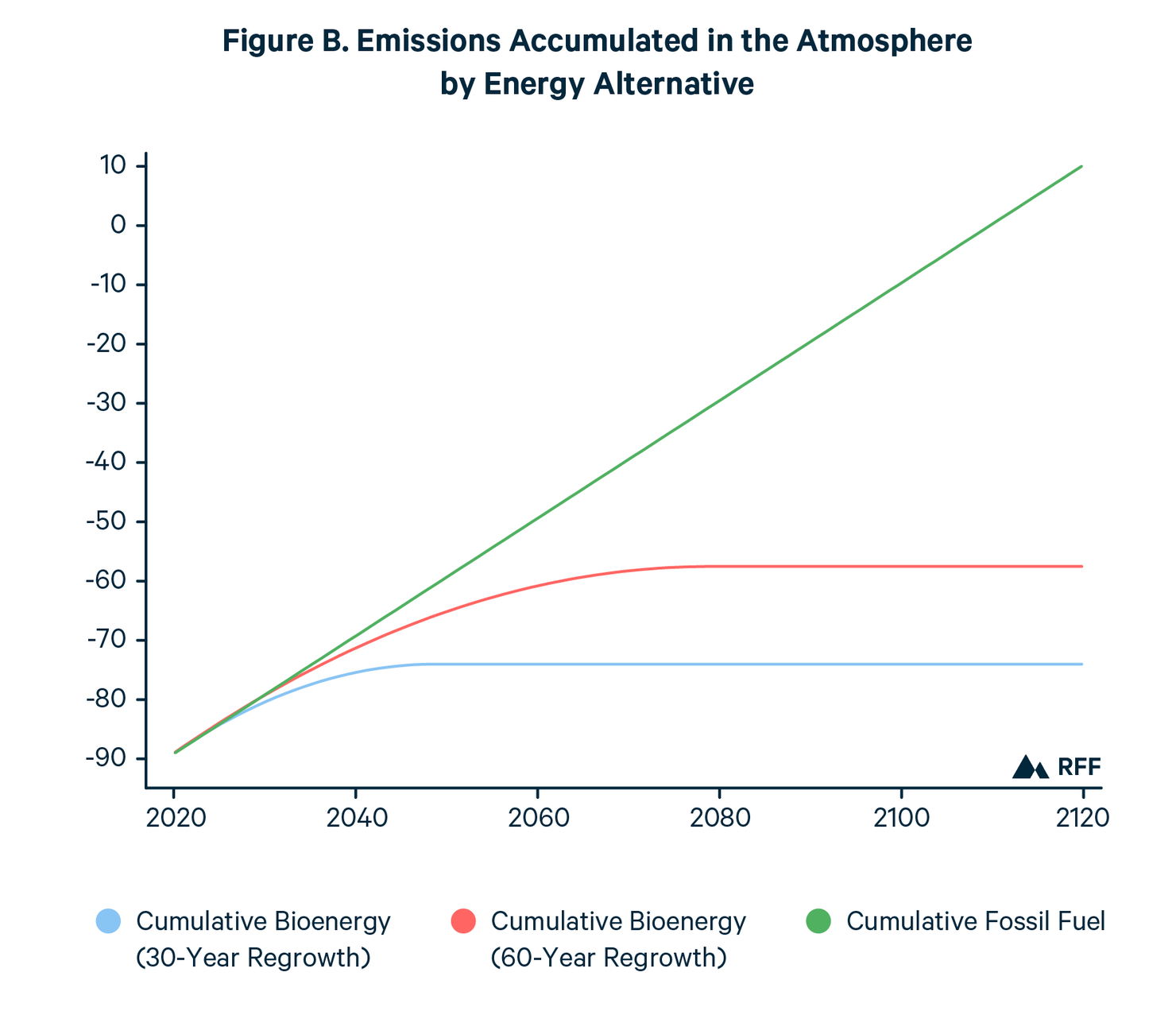
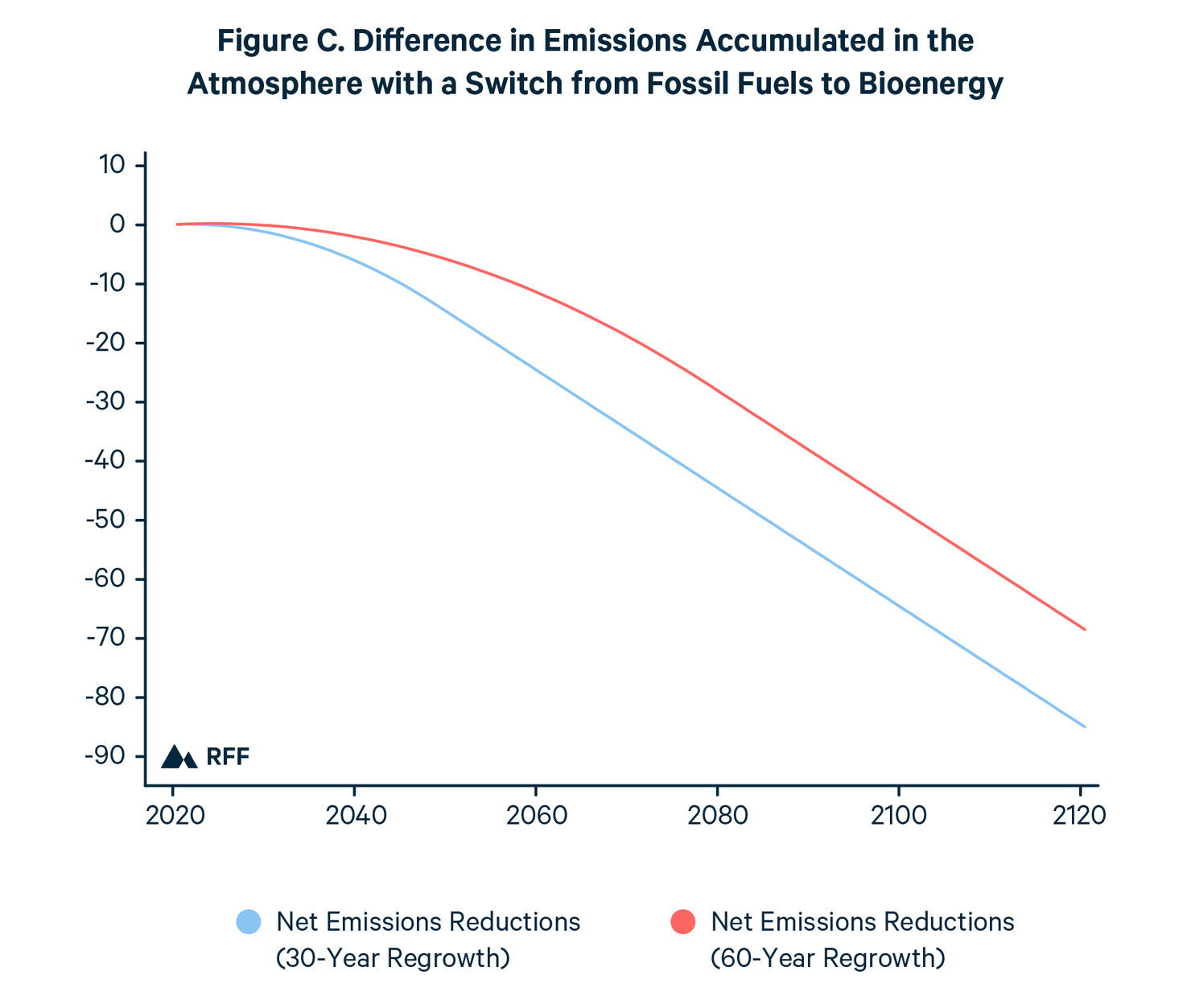
The case study illustrates how using bioenergy would reduce the atmospheric concentration of greenhouse gases compared to using fossil fuels (even without accounting for market-driven changes in land use and management). Of course, a more realistic example would account for wood waste feedstocks and the impacts of other market responses related to expanded forest bioenergy demands. In response to increased wood prices, the area of forest and the intensity of management would grow, further increasing the carbon captured by the forest sector.
Key Observations for a Closed Carbon Cycle:
- While fossil fuel emissions accumulate in the atmosphere, bioenergy emissions reach a steady-state level that represents the carbon flowing between the atmosphere and living biomass.
- During the initial phase of bioenergy use—the transition to a steady state—carbon emissions may equal or exceed the fossil fuel alternative. In this case, net emissions reductions accrue beginning at year 5.
- After the transition to a steady state, the emissions benefits of bioenergy accumulate steadily over time at the rate of avoided fossil fuel emissions.
Acknowledgments
The authors would like to thank Roger Ballentine, Francisco Aguilar, Bob Abt, and Brent Songhen for their helpful review and feedback.