Investment Tax Credits for Hydrogen Storage
An overview of hydrogen, benefits for energy storage, opportunities for/importance of decarbonization, and necessary infrastructure, all from the perspective of the tax credit.
Hydrogen in the Energy Storage Tax Incentive and Deployment Act of 2019
The Energy Storage Tax Incentive and Deployment Act of 2019, introduced by Representative Mike Doyle as H.R. 2096 and by Senator Martin Heinrich as S. 1142, would have extended the 30 percent energy investment tax credit to energy storage technologies, “equipment which receives, stores, and delivers energy.” With hydrogen among the technologies specified, the act would have had the potential to (1) motivate the utilization of hydrogen to store and deliver power and (2) reduce carbon dioxide (CO2) emissions from hydrogen production. This tax credit was not included in the 2020 non-defense appropriations package. However, to inform future versions of this legislation, we have prepared this issue brief to describe hydrogen’s role in energy storage, production methods and costs, and infrastructure.
Hydrogen Overview
Hydrogen (H2) is of interest because of its wide range of applications and the fact that its combustion generates no CO2 (although its production can generate large quantities of CO2, depending on the method). Currently, hydrogen is produced on a massive scale for the industrial sector, with global use of approximately 70 million metric tons per year (equivalent to the energy content of 50 percent of annual US gasoline consumption). Hydrogen is primarily used for the production of ammonia and the processing of petroleum fuels. While the proposed tax credit was limited to energy storage, hydrogen has the potential for extensive use as a decarbonized fuel—in transportation, industrial heat, commercial and residential space heating, and combined heat and power. For industrial heat, hydrogen is one of few options that provide the necessary high temperatures and have the possibility of low emissions.
Benefits for Hydrogen for Energy Storage
Wind and solar power make up an increasingly significant share of US electricity generation; they have gone from being negligible energy sources in 2000 to 10 percent of US power generation today, and they may contribute up to 31 percent of power by 2030 if their respective tax credits are extended. [1] Therefore, energy storage is increasingly important to limit the costs of managing intermittent generation and avoid wasting energy that cannot be used at a particular time (known as curtailment).
A challenge with wind and solar power is that their output varies—not only throughout the day but also over weeks and months. While lithium-ion batteries are a good option to frequently store and discharge energy for several hours, their viability decreases as storage durations increase and occur less regularly. Storing energy in a battery requires expensive capacity; it is harder to cover the fixed costs when that capacity is used less frequently. In contrast, once it has been produced, hydrogen can be stored relatively cheaply and then used over any time frame. To generate electricity, hydrogen can be used in either a combustion turbine or a fuel cell. Compared with the other options for long-term energy storage, such as pumped hydropower and compressed air, hydrogen has the advantages of having fewer locational constraints and some ability to use existing natural gas pipelines and related infrastructure (explained below).
Potential to Decarbonize Hydrogen Production
Although the combustion of hydrogen does not generate CO2, the current production processes are very CO2-intensive. Global hydrogen production accounted for 830 million tons of CO2 in 2017, an amount equal to 2.2 percent of global fossil CO2 emissions and more than the emissions from Germany. [2] Over 95 percent of current hydrogen generation is from steam methane reforming (SMR), producing what is known as “gray hydrogen,” or from gasification of coal, producing “brown hydrogen.” A promising pathway to decarbonized hydrogen is SMR or gasification combined with carbon capture and sequestration (CCS), producing “blue hydrogen.”
Less than 5 percent of hydrogen is generated from the electrolysis of water, and this process costs three to six times more than those of SMR. [3] A large enough investment tax credit for hydrogen produced using electricity would promote a method with the potential for reduced CO2 emissions. The amount of CO2 emissions depends on the source of power. If the electricity to power this process comes from renewables or nuclear power, the hydrogen would be decarbonized and known as “green hydrogen.” Only a tiny percentage of electrolyzed hydrogen is produced this way, but there is rising global interest in green hydrogen production. The green hydrogen project pipeline for the next five years is 12 times larger than all the green hydrogen capacity installed since 2000. [2]
Figure 1. Hydrogen Production Processes
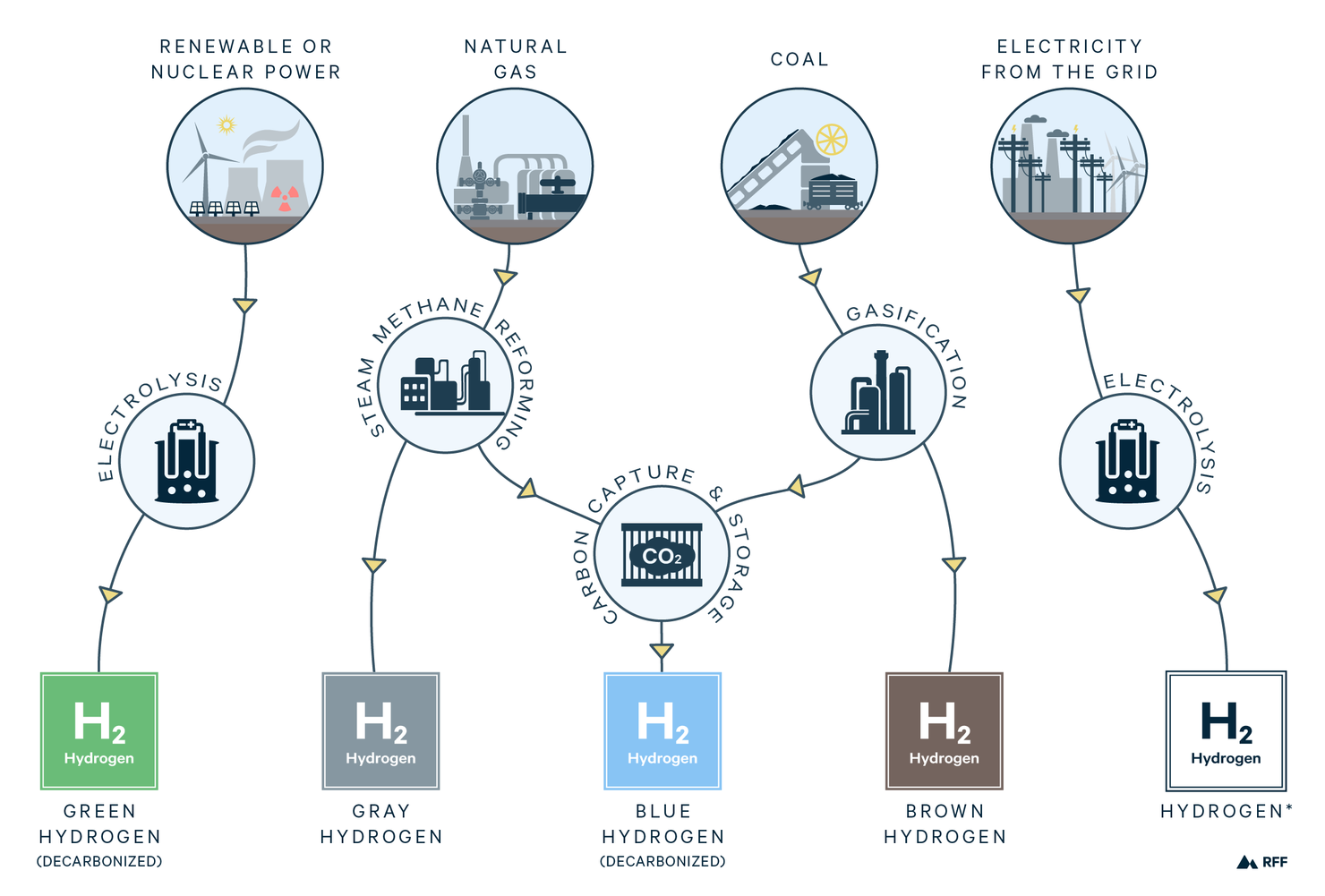
With increasing wind and solar power on the grid, there will be hours when electricity has a price of zero (or even be negative). This has led to the false hope that electrolysis could be powered by free (and green) electricity. Although early electrolysis capacity could receive some hours of zero-priced power, as more electrolysis capacity (and other demand) is added, the amount of “free” electricity would diminish. Furthermore, if electrolysis operates only during “curtailment” hours, it will not operate long enough to offset its high capital costs.
A tax credit for hydrogen produced using electricity has two potential deficiencies (apart from whether the credit itself is large enough). First, the tax credit would be available to electrolysis projects regardless of the source of power. Therefore, the hydrogen produced could result in substantial emissions. Second, the tax credit could potentially be used by SMR projects with reactors using electric rather than gas-fired heating (SMR requires steam at around 900°C). Although electrified SMR would have lower emissions than combustion-based SMR, without CCS the hydrogen produced would have higher emissions than its alternative, directly using natural gas for power generation.
While SMR with CCS (blue hydrogen) would not be eligible for a tax credit based on electrolysis, such projects would be eligible for the tax credits under Section 45Q. However, 45Q has a deadline of December 31, 2023 for starting construction, so without an extension, blue hydrogen projects that commence construction afterwards would not receive any federal support. This suggests that perhaps some coordination would be needed between a hydrogen ITC and 45Q.
Hydrogen Infrastructure
While safety is a common concern with hydrogen, with appropriate technology, it can be transported and used comparatively safely. In fact, in some respects, it is safer than natural gas. Hydrogen disperses more quickly and is nontoxic. On the other hand, hydrogen has a less visible flame than natural gas and can ignite at lower concentrations, so more careful leak and flame detection are needed.
Figure 2. Infrastructure Options for Hydrogen Power
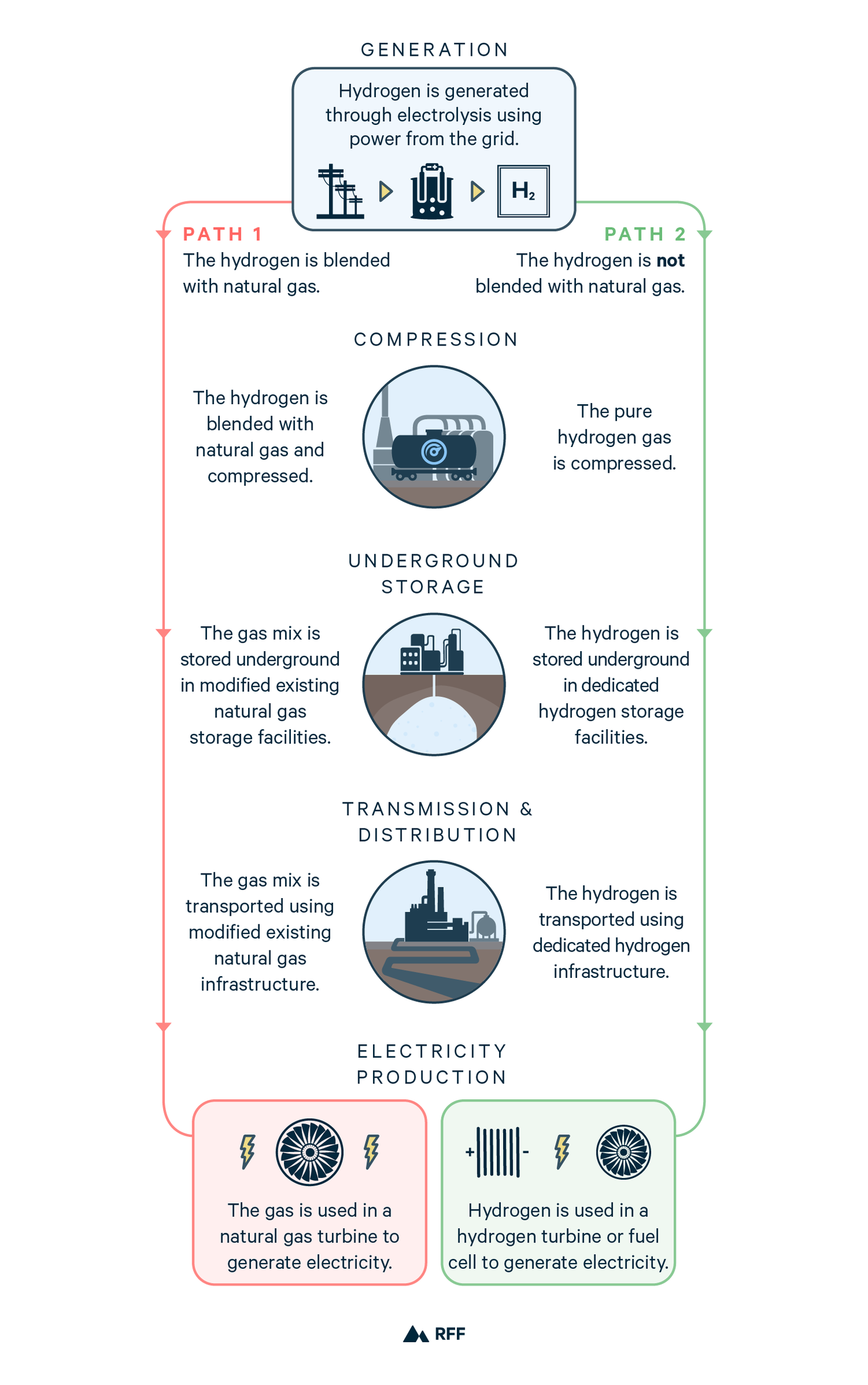
As shown in Figure 2, hydrogen can make use of existing natural gas infrastructure, such as pipelines and combustion turbines, but only if it is blended with natural gas. However, hydrogen is less dense than natural gas, requiring greater compression, and it can cause steel infrastructure to become brittle. Therefore, as the percentage of hydrogen in the blend increases, equipment modifications and then specialized infrastructure become necessary.
Hydrogen can be stored under pressure in salt caverns and depleted oil and gas fields. Storage does impose costs and has some geographic constraints, but suitable geologic features can be found in various regions of the United States.
Finally, we note that the water usage for electrolysis—even on the scale of natural gas consumption—would be minimal relative to the amount of water currently used to cool thermal power plants in the United States.
What’s Coming from Us: Hydrogen for Industrial and Power Uses
We will later publish a more detailed report on how a tax credit for decarbonized hydrogen modeled on 45Q for CCUS could be efficiently designed to reduce emissions in the industrial and power sectors.
References
- Larson, J., W. Herndon, H. Kolus, and G. Hiltbrand. 2019. “Can Tax Credits Tackle Climate?” Rhodium Group. https://rhg.com/research/can-tax-credits-tackle-climate/.
- Wood Mackenzie Power & Renewables. 2019. “Green Hydrogen Production: Landscape, Projects and Costs.” https://www.woodmac.com/our-expertise/focus/transition/green-hydrogen-production-2019/.
- Friedman, S. J., Z. Fan, and K. Tang. 2019. “Low-Carbon Heat Solutions for Heavy Industry: Sources, Options, and Costs Today.” New York: Columbia University, Center on Global Energy Policy.