Medium- and Heavy-Duty Vehicle Electrification: Challenges, Policy Solutions, and Open Research Questions
In this report, RFF researchers lay out the major challenges of and potential policy solutions to medium- and heavy-duty vehicle electrification.
Abstract
Medium- and heavy-duty electric vehicles (MHDEVs) are a promising solution to decarbonizing our transportation sector. Not only is the technology advanced, but multiple stakeholders are generating momentum for the adoption of clean vehicles. However, many challenges remain. In this paper, we describe the economics of adoption, electric vehicle logistics and operations, vehicle manufacturing, required infrastructure investments, barriers to an equitable transition, and both positive and negative externalities. We also discuss low-carbon alternatives to diesel vehicles. We next discuss policy solutions for the challenges (including both existing and potential policies). Finally, based on our literature review, we present open research questions whose answers would help resolve the challenges.
Executive Summary
Trucks and buses are disproportionately large contributors to air pollution and greenhouse gas emissions. Though only about 5 percent of total vehicles on US roads, in 2020 they accounted for approximately 26 percent of the transportation sector’s greenhouse gas emissions. Electric trucks and buses are a promising solution but face many hurdles. Based on a literature review, interviews, and learnings from a conference on electrification, this paper identifies major challenges, policies to address them, and open questions for further research.
Challenges
Costs to fleets. The total cost of ownership (TCO) of medium- and heavy-duty electric vehicles (MHDEVs) needs to be competitive with that of diesel vehicles. Their purchase price, however, is much higher, and charging station installation can exceed $100,000. Recharging costs depend on electricity tariffs and thus can vary by location. MHDEV maintenance costs are generally lower, but fleets may need specialized workers. Vehicles’ residual value depends on battery degradation and demand for used MHDEVs—factors that are currently unknown.
Logistics and operations. MHDEVs must be comparable to diesel vehicles in model options, range, recharge time, payloads, and maintenance. MHDEVs generally have ranges below 200 miles, versus more than 1,000 miles for diesel vehicles. Recharge times are substantially longer than diesel refueling, and fleets without depots need public charging stations. MHDEVs also weigh more, which may mean less payload. All these factors could increase their TCO relative to diesel.
Manufacturing challenges. Manufacturers of MHDEVs must create production tools and processes from scratch. As battery technology improves, each change necessitates new equipment and vehicle configurations. With low demand, manufacturers are uncertain about procurement; fleets are uncertain about manufacturers’ longevity. The small number of manufacturers in each vehicle class and use category gives makers market power to keep prices high. As sales grow, the pace of mining exploration and development for critical minerals may be restrictive.
Infrastructure requirements. MHDEV charging (which may exceed several MWs of demand for large fleets) could destabilize electricity distribution systems. Investments in the grid, transmission system, and generation capacity are required. Long-haul fleets without depots, as well as small fleets and owner-operators for whom charging stations are cost-prohibitive, will require midday, on-route fill-ups at public charging stations. Public stations can also necessitate investments in the grid, but MHDEV traffic flows, and thus loads, are unpredictable. To maximize environmental benefits, the new generation used for charging will need to come from renewable sources.
Equity. Small fleets will likely purchase MHDEVs in the secondary market and therefore not benefit from incentives for new-vehicle buyers. Small fleets also face bureaucratic and administrative hurdles because they lack the staffing to take advantage of incentive programs and optimize TCO after purchase.
Externalities. Adoption of MHDEVs can generate externalities, both positive and negative. MHDEVs reduce dependence on imported oil and can improve local air quality, but fleet owners are unlikely to internalize such benefits—or the social and environmental costs associated with charging. Research by one manufacturer can benefit others, yet these benefits are unlikely to be internalized, leading to suboptimal investment in R&D.
Alternative Technologies
Low-carbon alternatives to MHDEVs may be needed for certain applications. Vehicles with hydrogen fuel cells or hybrid technology would have shorter refueling times and lighter weight (i.e., less payload reduction). Biodiesel fuel is another alternative; it doesn’t require fleets to purchase new vehicles, and it has the potential for reducing emissions (though it can have unintended consequences, such as land-use changes).
Policy Solutions
One goal for policymakers is to increase MHDEV adoption by lowering manufacturing and operating costs. Some policies can leverage market power (e.g., electricity tariffs that incentivize managed charging); others lower prices directly (e.g., purchase incentives). A second goal is to maximize the economic, environmental, and social benefits of electric vehicles. Here, we list policy options to help increase adoption while maximizing benefits to society.
Lowering upfront costs. Subsidies can promote electrification by lowering costs for purchase and charging infrastructure. Given the importance of fast charging for commercial fleets, support for Level III charging may be crucial. Incentives for manufacturers to add models (e.g., zero-emission vehicle credits in CAFE standards, electric vehicle manufacturing tax credits) would lower entry costs and encourage diversification (to limit market power). Fleet mandates could also help manufacturers predict demand and make procurement more efficient.
Managing operating costs. Lower operating costs would make MHDEVs more affordable, but reductions must not increase social costs. Policymakers have several options. Electricity tariffs can encourage fleets to reduce maximum demand (by charging more slowly) and peak-hour consumption (by charging off-peak). A low-carbon fuel standard and vehicle-to-grid contracts can earn extra revenue for fleets. Automated managed charging software allows fleets to respond to prices automatically. Co-located solar and storage can reduce fleets’ maximum demand and demand on the grid. Finally, higher diesel fuel prices can help smooth the difference in operating costs across vehicle types.
Improving on-route charging. The National Electric Vehicle Infrastructure Formula Program provides funding for charging stations, but more investment in low-traffic areas is needed. Green loading zones could provide dedicated MHDEV parking spaces with charging stations. Battery swapping would enable efficient exchange of a depleted battery with a fully charged one. Electric road systems could address the challenge of long charging times, particularly for MHDEVs without depots.
Dealing with externalities. The new generation capacity required to meet increased demand should be clean. Policies that promote clean electricity simultaneously with a clean transportation system include carbon taxes, renewable portfolio standards, and cap-and-trade programs. Incentives for co-located storage and solar and managed charging software could also help reduce emissions associated with charging.
Increasing adoption by small fleets. Technical assistance can help small fleets and operators in environmental justice (EJ) communities surmount logistical challenges. Subsidies should be tailored to small fleets and fleets in low- and moderate-income and EJ communities and include financing for managed charging software and co-located storage and solar—investments to help small fleets go electric while also reducing effects on the grid.
Open Questions
The uncertainties in the MHDEV market make it challenging to craft policies that promote efficient, effective, and equitable outcomes. Our work has identified open questions for the research community.
Vehicle use and ownership. Answers to these questions can inform policies to encourage fleet adoption.
- How do purchase and charging station subsidies affect the TCO of MHDEVs?
- What is the optimal level for electricity tariffs to change the TCO and incentivize managed charging?
- What is the depreciation rate for MHDEVs? The residual value of used electric vehicles? How will truck purchase and ownership patterns shift in response?
- How much would co-located storage and solar reduce the TCO?
- What payment and contract structures for vehicle-to-grid would maximize cost-effectiveness?
- How would higher diesel prices affect MHDEV adoption?
Manufacturing and supply chains. Answers to these questions can help policies accelerate the transition while promoting market efficiency.
- Would R&D investments lead to greater entry, product offerings. and innovation?
- What is the value of technological spillovers in the MHDEV sector?
- How does market power affect truck prices?
- How are supplies and costs of critical minerals constraining development of MHDEVs?
Public charging stations. Answers to these questions could make charging station investments more efficient and equitable.
- How do public charging investments affect adoption decisions and interact with other incentives?
- What are the network issues for these investments and the charging market?
- How can public charging investments maximize benefits to EJ communities?
Table 1 summarizes the challenges to the MHDEV transition, possible policy solutions, and open questions.
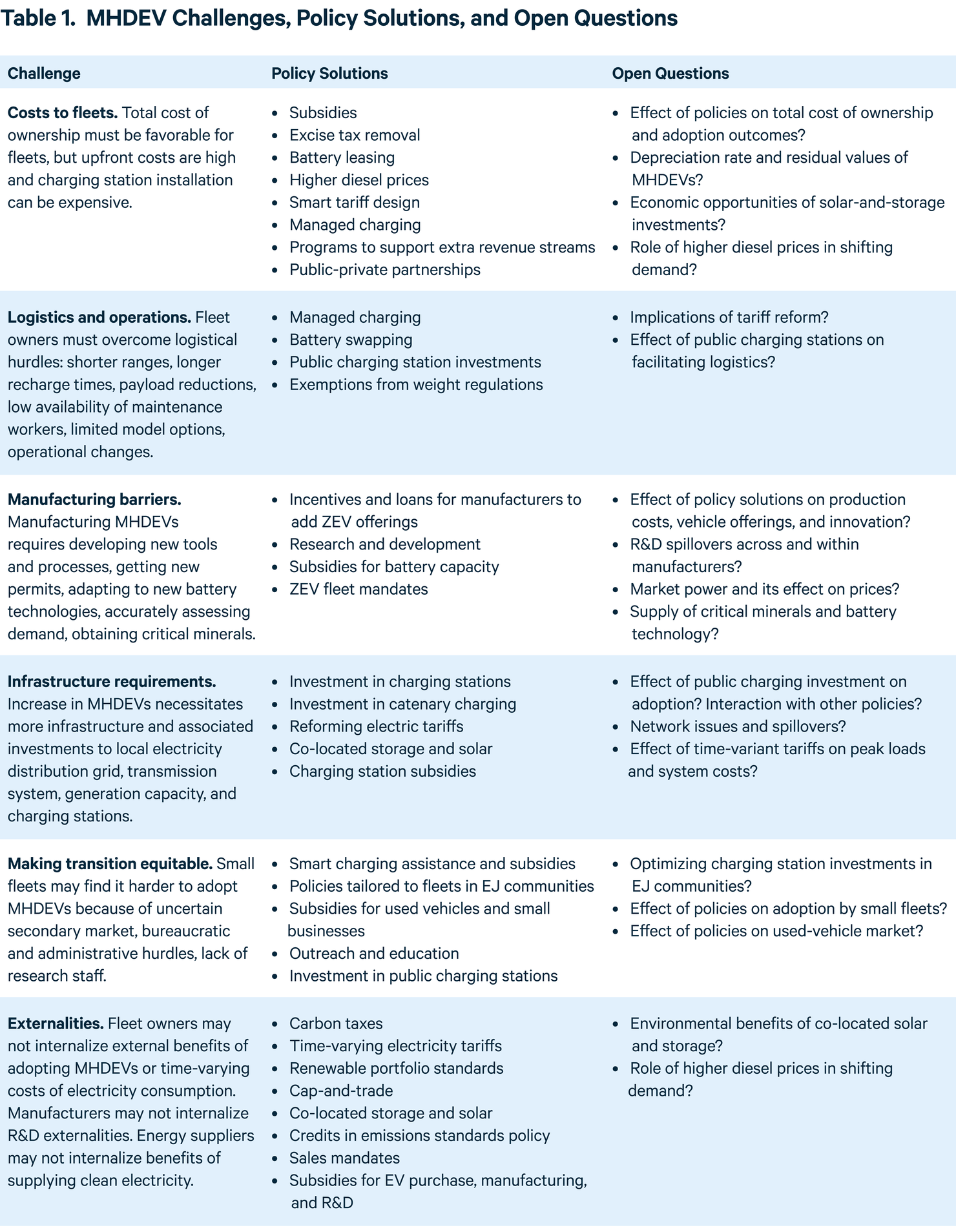
1. Introduction
Medium- and heavy-duty vehicles are an important part of the economy. They provide long-haul and local delivery, transport people and products, collect garbage, support construction, and much more. Trucks and buses also are a significant and disproportionate contributor to local air pollution and greenhouse gas emissions. In fact, though they represent only a small fraction of total vehicles on the road (approximately 5 percent in the United States Bureau of Transportation Statistics, “Number of US Aircraft, Vehicles, Vessels, and Other Conveyances.”), in 2020 they contributed approximately 26 percent of greenhouse gas emissions coming from the transportation sector US Environmental Protection Agency, “Fast Facts on Transportation Greenhouse Gas Emissions.”. Furthermore, COVID19 led to an increase in deliveries, a trend shift that is likely to continue increasing the contribution of MHDVs to transportation sector emissions.
Truck and bus emissions tend to disproportionately affect environmental justice (EJ) communities, which are located closer to major roadways and the warehouses and depots where the vehicles are housed. Reducing emissions from this sector will not only improve air quality and reduce the transportation sector’s contribution to climate change, it will also improve health and well-being in communities historically affected by transportation pollution.
Decarbonizing trucks and buses—to reduce both greenhouse gases and local air pollution—is an important goal for improving welfare, health, and well-being across the nation, as well as mitigating climate change. Vehicle electrification is a promising solution because the technology has now developed to such a degree that many use cases could shift to electric; electric vehicles are on the market and have been proven to work. Many challenges remain, however. In this paper, we describe the challenges, study what policies have been or could be leveraged to address these challenges, and identify open questions and gaps in knowledge that can contribute to policymaking.
MHDV electrification is one of several options available to decarbonize the sector. We choose to focus on this solution for two reasons.
1.1. Momentum across Stakeholders
First, stakeholders are generating momentum for truck electrification. At the federal level, the United States is a part of the Global Memorandum of Understanding (MOU) on Zero-Emission Medium- and Heavy-Duty Vehicles. This MOU commits participating countries to work together to achieve net-zero carbon emissions by 2050 by setting a goal of 100 percent zero-emission new truck and bus sales by 2040, with an interim goal of 30 percent zero-emission vehicle (ZEV) sales by 2030. The Inflation Reduction Act (IRA) of 2022 shows commitment to the cause and provides billions of dollars in funding for MHDV electrification through subsidies and grants for vehicle purchases, charging station investments, and advanced vehicle manufacturing.
Some states have their own plans to achieve electrification. New York school buses must be electrified by 2035. Both California and New York have set goals of achieving 100 percent zero-emission truck and bus sales by 2045. In a multistate MOU facilitated by NESCAUM Northeast States for Coordinated Air Use Management, an association of air quality agencies in New England, New Jersey, and New York., 17 states and Washington, DC, have agreed to achieving 30 percent zero-emission truck and bus sales by 2030.
The trucking industry, both manufacturers and fleet operators, has also set its sights on electrification. Tesla delivered its first battery electric heavy-duty semi truck with a range of 500 miles on a single charge. With a pilot partner, Volvo is building a national network of public heavy-duty charging stations. New companies, such as Rivian and Proterra, have emerged that exclusively manufacture MHDEVs. Many diesel vehicle manufacturers are releasing electric options and have their own goals for electric sales; Volvo, for example, is aiming for 50 percent electric sales by 2030. Major companies and fleets, like USPS, PepsiCo, and Amazon, are putting in preorders for thousands of electric trucks and delivery vans.
The electric utilities in this country have joined the push toward MHDV electrification as well. Many utilities have started pilots and projects to ensure a smooth transition to vehicle electrification so that the increase in demand from these vehicles can be met cost-effectively.
Finally, communities are demanding cleaner air and view MHDEVs as important for achieving this goal. Electrification of this sector will improve the health and well-being of communities, particularly disadvantaged and low-income communities, which tend to be closer to major roadways, warehouses, and depots and are therefore disproportionately affected by transportation pollution. See, for example, public support for electric school buses by WeACT for Environmental Justice (a community organization based in West Harlem, New York City); and a call for full truck electrification in California by a group of more than 70 organizations, including local environmental justice, labor, and health groups.
1.2. Technical Feasibility
Second, electrification is feasible. The potential for decarbonization arises from the ability of electric vehicles (EVs) to convert energy into movement at a much higher efficiency rate than diesel vehicles. Furthermore, electric technology has rapidly advanced. With growth in light-duty EV adoption and manufacturing, battery technology has improved significantly, leading to cost reductions of 97 percent over recent decades. The price of batteries has declined by 97 percent in the last three decades. These advancements can bring cost savings to the MHDV sector as well.
The collective effort to electrify the MHDV sector and the technological potential of these vehicles bode well, but the transition must be effective, efficient, low-cost, and equitable. An optimal transition starts with a full understanding of the challenges faced by utilities, manufacturers, fleets, and communities. Policies can then be crafted to target and reduce the impediments. Based on an exploration of the existing literature, multiple stakeholder interviews, and learnings from a conference panel dealing with MHDV electrification, The panel was part of Energy Insights (2022), a conference hosted by Resources for the Future and funded by the Alfred P. Sloan Foundation. this paper identifies the challenges and the policies that can address them, and also considers the open questions where further research is needed.
2. Challenge #1: Making the Economics of MHDEV Adoption Work
The first challenge is making the total cost of ownership (TCO) favorable to adoption. The TCO comprises the initial purchase cost of a vehicle, fueling and maintenance costs over the ownership period, and the vehicle’s residual value. An investment in MHDEVs involves a high upfront cost because of high vehicle prices and charging infrastructure installation; the economic benefit of adoption comes later, through lower fuel and maintenance costs. To make the TCO favorable to adoption of MHDEVs, policymakers must understand how this trade-off affects the purchasing decision.
2.1. Upfront Costs
Vehicle price. MHDEVs are substantially more expensive than their diesel alternatives. For example, the average price of a Class 8 electric truck in 2020 exceeded $300,000, compared with the $117,430 average price of a diesel truck in 2018. https://www.act-news.com/news/calculating-tco-for-medium-and-heavy-duty-evs/ High MHDEV prices can be prohibitive for fleets and can result from several factors, including high battery costs, start-up costs associated with the development of new EV models, and truck manufacturers’ market power, which may be high because of the limited commercial product offerings in the industry.
Charging station installation. For fleets that return to their depots at the end of their shift, investing in MHDEVs requires the installation of a charging station on site. Fleets that do not return to depot and single-vehicle owners are unlikely to find charging station investments optimal and thus will rely on public charging stations that serve MHDEVs. The decision of which charger to purchase is not trivial: there is a clear trade-off for the fleet owner between charger cost and speed of charging. Low-capacity chargers can be significantly less expensive but their longer changing times give the fleet less flexibility: they may not fully charge a large battery during the vehicle’s time at the depot. Higher-capacity chargers provide more flexibility and can even handle multi-vehicle charging. With the cost of the charger itself and installation costs (which vary), high-capacity chargers can exceed $100,000. https://reglobal.co/how-much-does-electric-vehicle-charging-infrastructure-in-the-us-really-cost/#:~:text=For%20example%2C%20for%20DCFCs%2C%20while,to%20EV%20charging%20infrastructure%20deployment
Further, the long lead time for charging infrastructure and grid upgrades could function as a cost because fleet owners must invest upfront for a payoff years down the line, and it adds uncertainty to a fleet’s decision to electrify. Thus, in choosing to go electric, the fleet must consider not only the purchase price of the vehicle but also the cost of the charging equipment and the installation lead time. The total cost of ownership can rapidly exceed that of a diesel alternative.
2.2. Operating Costs
Fuel cost. A 2021 survey by ATRI found that motor carriers and owner-operators incurred, on average, fuel costs of about $0.4 per mile, accounting for 22.5 percent of their per mile operational costs (Leslie and Murray 2022). Fuel costs add up over the vehicle’s lifetime, thus heavily affecting the TCO. The competitiveness of MHDEVs compared with their diesel alternatives depends on the relative cost of charging versus fueling. Relative prices may matter even more for those who are buying low-emission vehicles. It is therefore important to understand how electricity tariffs work for commercial businesses.
The electricity cost incurred by fleets generally includes two components: a volumetric (per kWh) charge, based on the amount of electricity the fleet consumed for the month, and a demand charge, based on the fleet’s maximum kW demand. Both volumetric and demand charges can be structured to fluctuate over the course of the day; by charging customers higher prices during more congested hours, these time-variant tariffs better reflect the underlying costs associated with charging. Yet many times these charges do not fluctuate throughout the day. Flat tariffs are problematic from a cost-causation point of view, because they do not incentivize appropriate managed charging that can help keep system costs down in the long run (Stein, Spiller and Koehler 2020). Importantly, time-variant tariffs also create opportunities for fleets to save by shifting demands and consumption into low-cost hours; and because off-peak hours are often during the night, for many fleets, this timing aligns well with dwell hours given daytime operations.
Maintenance costs. Maintenance and repair costs of MHDEVs are expected to be lower because electric engines are simpler than internal combustion engines (ICEs) (Mitropoulos et al. 2017, Hunter et al. 2021). This difference can be a significant source of savings and can help lower the TCO of electric vehicles compared with ICEs. However, because the savings accrue over the vehicle’s lifetime, businesses that require short payback periods may not incorporate them into their TCO estimates. Maintenance costs are also likely to evolve over the lifetime of a vehicle. Although it is intuitive to infer that they increase with age and cumulative usage, the difference in lifetime maintenance costs of electric vehicles compared with ICEs is an open question.
Insurance costs. Anecdotal evidence suggests that fleets considering EVs are facing higher insurance costs; This is on top of higher insurance costs across the trucking industry more broadly; major settlement cases from accidents have driven up rates. this may be due to new and unfamiliar technology, overall higher purchase costs, and higher costs of repair after accidents. https://www.moneygeek.com/insurance/auto/electric-vehicle-insurance-costs/
Though many studies have found favorable TCOs for MHDEVs, the input assumptions significantly affect the comparison (Burnham, et al. 2021, Hunter, et al. 2021, Ledna, et al. 2022). Given the trade-off between high upfront costs and low operating costs, the TCO of MHDEVs for the fleet owners ultimately depends on how much these trucks are used; a truck that is driven more miles would have a more favorable TCO.
3. Challenge #2: The Fleet Perspective: Logistics, Operations, and Vehicle Adoption
Fleets expect MHDEVs to be comparable with diesel vehicles in range, payload, availability and training of maintenance workers, and model options. Fleets also need to account for increased recharge time in their logistical considerations. In this section, we describe the logistical roadblocks fleets face when deciding whether to invest in electric vehicles.
3.1. Range
The current available range for electric trucks is less than 200 miles on a single charge Data on electric range for Class 8 trucks come from Price Digests.—much shorter than the range of comparable diesel vehicles, which (with two 150-gallon tanks) can go 2,000 miles without refueling. https://www.freightwaves.com/news/how-many-gallons-does-it-take-to-fill-up-a-big-rig#:~:text=How%20far%20can%20a%20big,consumption%20rate%20of%206%20mpg Technological improvements in batteries have allowed for longer ranges: the new Tesla Semi, for example, is said to reach 500 miles on a single charge. https://www.visualcapitalist.com/every-electric-semi-truck-model-in-one-graphic/#:~:text=This%20presents%20challenges%20for%20long,promises%20up%20to%20500%20miles For most long-haul fleets, the market has not evolved sufficiently, but for trucks that travel 100 to 200 miles (Moultak et al. 2017), fleet operators have more electric options.
The actual range of MHDEVs may differ from the manufacturer’s reported range, depending on factors such as driving style, temperature, and hill grade. Although the observed mileage of ICE vehicles can also differ from that reported by manufacturers, online forums such as fuelly.com host user-reported estimates of models’ MPG. Because MHDEVs are still not widespread, uncertainty about their range and range anxiety may cause a fleet operator to look for vehicles whose advertised ranges exceed its trucks’ actual daily mileage requirements; because vehicle purchase prices increase with range, this would increase the firm’s expected TCO, thereby potentially discouraging electrification. Furthermore, this concern may cause fleets to look for vehicles with ranges that are not commercially available, thus delaying their transition to electric. Importantly, 65 percent of medium-duty trucks and 49 percent of heavy-duty trucks drive short enough distances to electrify with currently available models (Lund, et al. 2022).
3.2. Recharging Time
Recharging MHDEVs takes far longer than filling a diesel truck’s tank. Charge times for some heavy-duty trucks can be up to nine hours (Moultak et al. 2017), though faster chargers can take significantly less time. MHDEVs that have a depot and overnight dwell periods will incur no opportunity cost of time, but the fleet owner must install a charger. The owner then faces a trade-off: paying a large amount for a high-speed charger or spending less on a slower charger.
Not all fleets can rely on recharging at the depot. In fact, almost 50 percent of MHDVs are owned by independent owner-operators (according to a recent American Trucking Association study https://bookertrans.com/what-percentage-of-truckers-are-owner-operators), who will be more likely to recharge on-route than install an expensive charging station at home. Furthermore, long-haul trucks, even if owned by large fleets, will require on-route charging, as will transit buses in large cities with long routes. Thus, the percentage and number of MHDEVs requiring public charging will vary but will likely be high in certain locations.
Recharging on-route raises two issues. First, the fleet must ensure that a public charging station is available (or alternatively, invest in on-route charging; for example, the New York City transit authority partnered with the New York Power Authority to invest $39 million in 50 on-route overhead chargers for city buses https://new.mta.info/press-release/mta-announces-plans-increase-number-of-electric-buses-purchased-2021). Second, the opportunity cost of the charging time adds to the total operating cost for the fleet. Today’s paucity of public charging stations for MHDEVs is a high hurdle for fleets that are not housed in depots. It also poses a challenge in optimizing the investment in building the network. Siting of stations needs to begin with well-traveled routes, and the stations themselves will require special design considerations for space and speed. Once public charging stations are more widespread and accessible, however, electrification could double (Liimatainen et al. 2019).
3.3. Payload Reduction
The high density of batteries generally makes an MHDEV heavier than its diesel equivalent Apart from the battery, an electric truck is actually lighter than its diesel alternative (Phadke et al 2021)., and the payload may need to be reduced to compensate for the extra weight (Phadke et al. 2021). The extent to which the payload needs to be reduced is unclear, however, and likely depends on several factors, such as fleet operations and vehicle type. For example, most trucks do not maximize their payload. MHDVs currently carry a 70 percent average payload, and a battery-powered vehicle on average is restricted to 80 percent of a usual diesel truck’s payload (Mareev, Becker and Sauer 2017). Some trucks, such as delivery vehicles, are packed based on volume; capacity, not weight, is their main payload limitation. For some use cases and vehicles, then, payload weight reductions may be only about 5 percent (Phadke et al. 2021). For larger trucks that move heavy freight or trucks that run at high cargo capacity, payload capacity reduction will be a larger concern, since it can directly affect the revenues from shipments. One study warns of a reduction in cargo capacity for vehicles that travel more than 500 miles because of the size of the battery (Verbruggen et al. 2018).
3.3. Vehicle and Infrastructure Maintenance
Diesel fleets usually employ maintenance workers to ensure that the trucks continue to function properly. Although the costs will be lower for MHDEVs, the vehicles and charging stations will still require maintenance (Fenton and Kailas 2021). Fleets will likely need to invest in retraining their fleet managers and maintenance staff or hire new experts.
4. Challenge #3: Manufacturing Challenges
MHDEVs are significantly more expensive than their diesel counterparts. Understanding the manufacturing challenges behind the high prices can inform policies that will be effective in bringing down costs and reducing the vehicles’ total cost of ownership. The challenges, identified through interviews with experts and major MHDEV manufacturers, are low demand levels, idiosyncratic manufacturing requirements, rapidly changing battery technology, heterogeneity across vehicle use cases, and supply chain restrictions.
4.1. Low Demand and Economies of Scale
In the nascent MHDEV market, low demand creates challenges in terms of economies of scale. MHDVs are manufactured generally on demand rather than in large batches, as in the light-duty vehicle market. Uncertainty about demand over the coming years increases costs because manufacturers have to pay higher prices for smaller orders of parts and materials. Certainty has the opposite effect: large orders from large fleets such as Amazon can give manufacturers the confidence to purchase large volumes of parts and materials at lower prices.
Low demand levels leading to low manufacturing output also create a risk that can reduce fleets’ incentive to adopt MHDEVs. When fleets purchase MHDVs, the manufacturer generally provides servicing support throughout the lifetime of the vehicle. If a vehicle might be pulled from the market or, in the case of start-ups, if the manufacturer itself might go out of business, the purchaser incurs a risk. For example, Chanje, a company that produced electric delivery vans, went out of business in 2021. Ryder, a fleet management company, had purchased 125 electric vans from Chanje but received only 25 before the company folded; Ryder is now suing the company for almost $4 million.
Fraudulent actions on the part of some start-ups also create a sense that purchasing vehicles from a new manufacturer is risky. For example, when Nikola Corp., an all-electric truck manufacturing company, was launched in 2014, many all-electric original equipment manufacturers (OEMs) were going public through special-purpose acquisition companies, allowing these companies to market themselves given projections of future performance. Through the use of fake videos (in one, a nonfunctional truck was rolled rather than driven down a hill) and false claims, the company was able to acquire more than $500 million in investment. The fraud was uncovered, and the company’s owner was found guilty of fraud in October 2022. https://www.latimes.com/business/story/2022-10-14/head-of-zero-emission-truck-venture-found-guilty-of-fraud Though Nikola is still in operation, the aura of risk around new manufacturers may not only reduce the incentive to adopt these vehicles but also minimize the market competition benefit of new start-ups.
4.2. Idiosyncratic Manufacturing Processes
The manufacture of electric trucks and buses represents a huge change in industrial practices (Smith, et al. 2019, Qi, et al. 2022) because a different kind of automation is required. Manufacturers can’t just drop a big battery into the shell of a diesel vehicle; rather, they must create the tools and processes for manufacturing these vehicles from scratch. MHDEVs must achieve acceptable levels of torque and horsepower to allow them to climb hills and maneuver through city traffic (Galvin 2022). The electric technology eliminates the need for an engine cooling system (though battery cooling systems are still required) and transmission (Phadke et al. 2021). And given the weight of the battery, the vehicle’s weight must be reduced in compensation to avoid reductions in payload (see Section 3.3). The differences require a rethinking of the vehicle’s aerodynamics and the materials used in its frame to decrease drag and minimize weight.
Making MHDEVs therefore requires significant investment in manufacturing equipment, tools, and processes for both existing OEMs that specialize in diesel vehicles and start-up companies that build EVs only. OEMs have one advantage over start-ups: their knowledge base in building vehicles and understanding what truck and bus drivers want in comfort and cab configuration. Given current low demand levels, high fixed costs of entering the electric vehicle market are spread over small sales in the short run, further increasing the cost differential between electric and diesel trucks and buses.
4.3. Changing Battery Technology
Battery technology has changed rapidly: today’s batteries are denser, thus allowing for longer ranges in a smaller battery (Liimatainen et al. 2019). Each improvement, however, forces the manufacturer to purchase new equipment as well as reconfigure its approach to installing the battery in the vehicle, thereby increasing the cost of assembly. Given low demand levels, vehicle prices will likely stay high until manufacturers can spread these costs over greater numbers of sales.
4.4. Heterogeneity across Vehicle Use Cases
MHDEVs are challenging to build because the use cases are heterogeneous (Birky, et al. 2017, Smith, et al. 2019). For example, refrigerated trucks driven on highways look very much like a residential delivery box truck, yet have very different patterns of driving; thus, the batteries required to power them will vary given, for example, their differing ability to leverage regenerative braking. Truck manufacturers therefore tend to specialize in a class or use case. In Figure 1, we leverage data from the ZETI database (CALSTART 2022) and a national-level vehicle registration data set to plot total sales and number of vehicle use cases for manufacturers with MHDEV sales through August 2022. (Importantly, 42 other OEMs appear in the ZETI database but have zero observed sales, and both Smith Electric and Chanje are no longer in business.) The figure demonstrates that the majority of the manufacturers with observable sales specialize in only one use case. Exceptions include BYD Motors, Lion Electric, and Greenpower (though this company’s sales do not exceed 200 vehicles), but closer inspection shows that BYD Motors has mainly sold non-school buses (1,208 sales) and tractor trucks (336 sales), Lion Electric has sold only school buses, and Greenpower has mainly sold only non-school buses (180 sales).
Figure 1. US Sales and Vehicles
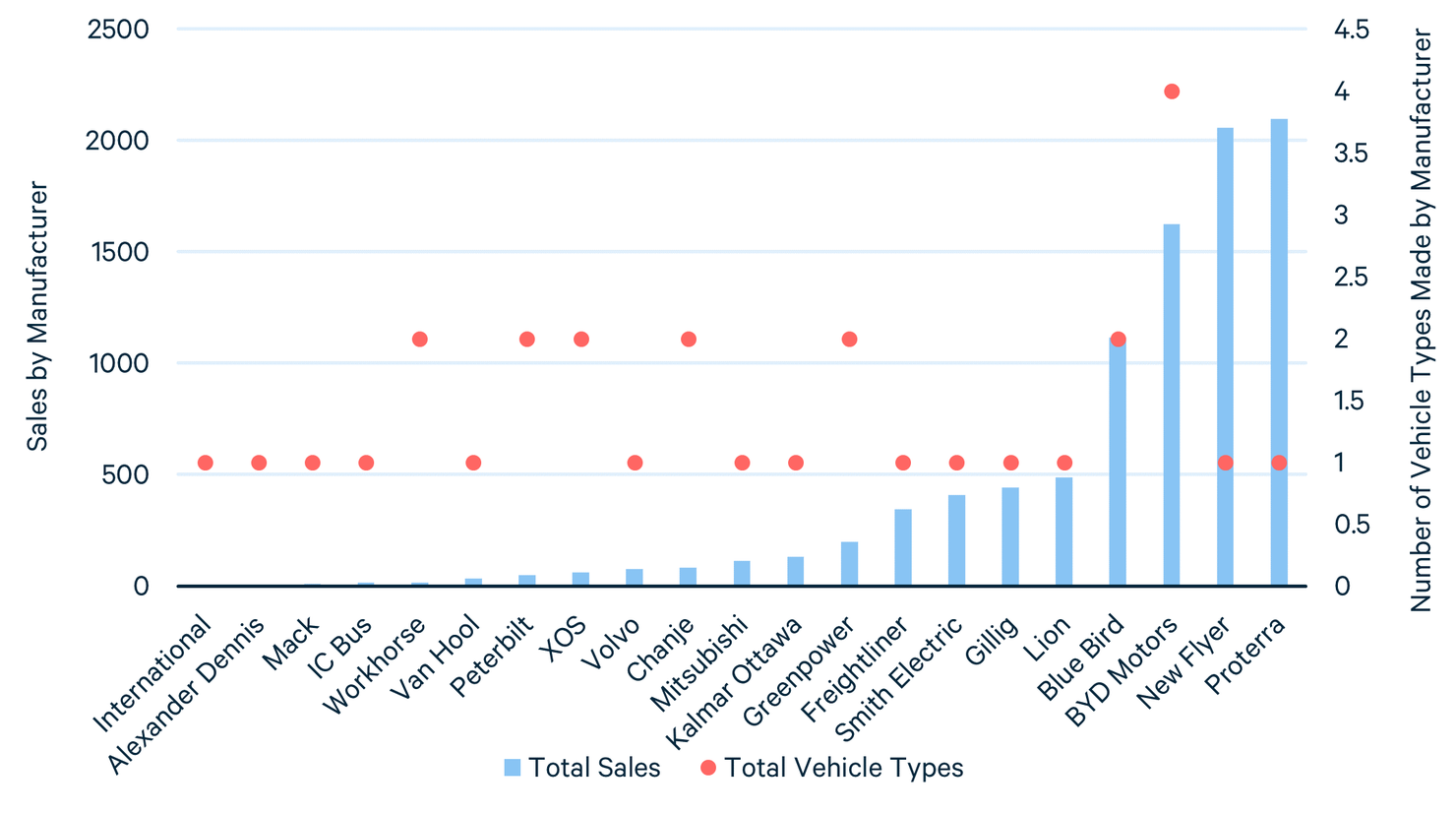
Note: Data come from ZETI database and a national-level vehicle registration data set.
Figure 2 plots the number of OEMs with sales above 200 vehicles within each category. It shows a significant concentration of OEMs within each use case and vehicle class. The non-school bus category has more competition (with six manufacturers, though Proterra, New Flyer, and BYD dominate the market) and the highest sales; in the majority of vehicle use types, only one manufacturer is selling that vehicle, with notably low sales.
Figure 2. Original Equipment Manufacturers and US Sales per Use Case
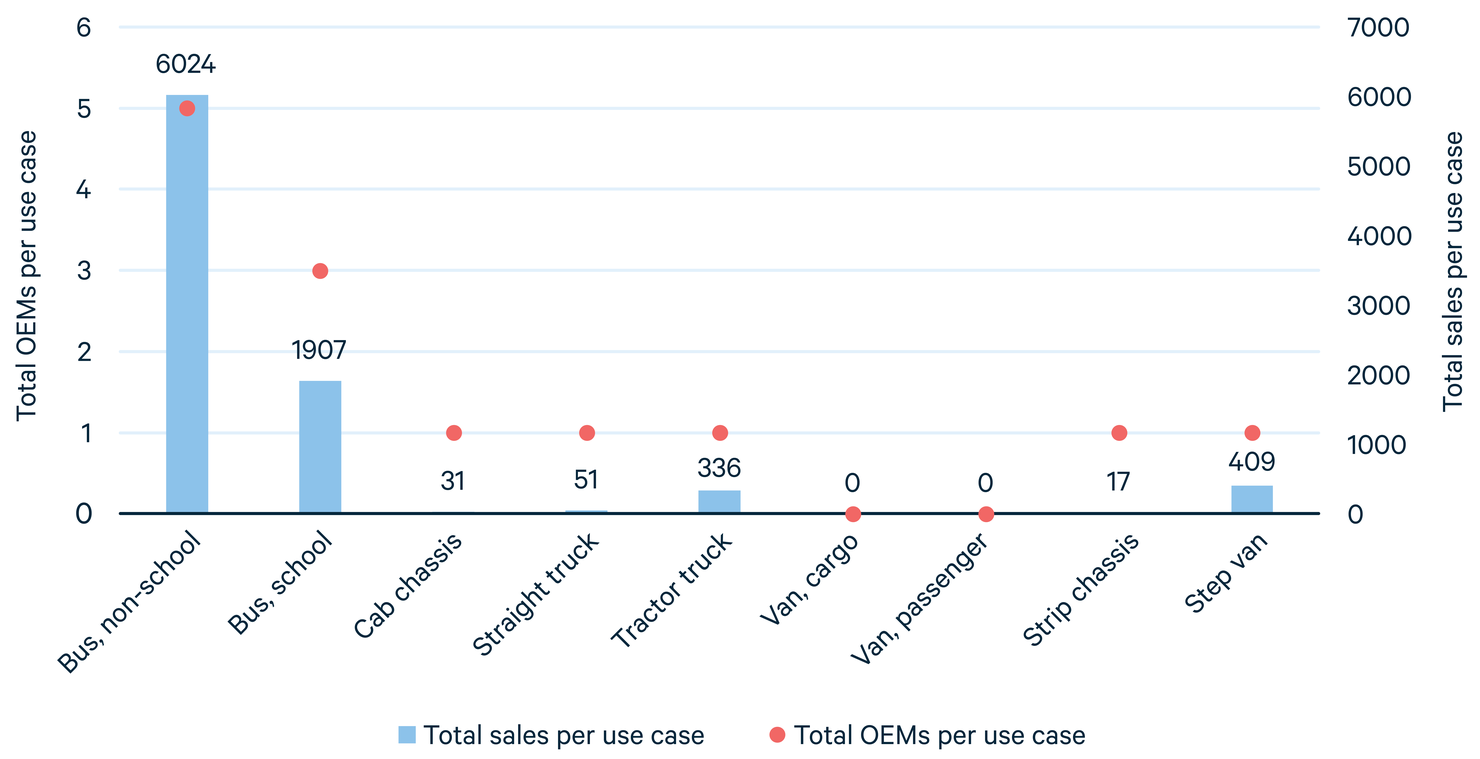
Note: Numbers reflect battery electric vehicle registrations in the first eight months of 2022 and OEMs with sales above 200 vehicles.
Because of the small number of manufacturers in each vehicle class and use category, market power is likely a factor in keeping prices high. Essentially, manufacturers facing little competition can pass through costs directly and inflate the purchase price.
4.5. Supply Chain Restrictions
A large constriction in the supply chain of MHDEVs is the critical minerals used to make the batteries. As vehicle demand has increased, so too has demand for these minerals. From 2021 to 2022, the price of lithium ion battery packs increased by 7 percent after more than a decade of cost declines (though some of this increase may have been due to inflation). https://about.bnef.com/blog/lithium-ion-battery-pack-prices-rise-for-first-time-to-an-average-of-151-kwh/
Bloomberg forecasts that as extraction picks up and refining capacity comes online, battery prices will begin to drop by 2024. However, MHDEVs require significantly more critical minerals than light-duty EVs, and as sales grow, demand for critical minerals may spur mining exploration and development.
5. Challenge #4: Infrastructure Investment Requirements
Electrification of the MHDV sector requires large batteries whose recharging raises electricity demand and consumption. For example, a fleet of 51 Class 7 and Class 8 vehicles in California could consume more than 4GWh of electricity per year, with maximum peak demand exceeding 4MW (Hamilton and Couch 2021)—almost as much as an outdoor sports stadium (about 5MW of peak demand). Many fleets are much larger, some with 500 vehicles. Investments in the distribution grid, the transmission system, and generation capacity will be necessary.
5.1. Local Electricity Distribution
MHDEV adoption will particularly affect the local electricity distribution system. The system that distributes electricity to homes and businesses is comprised of many pieces of infrastructure—large substations, underground or overhead wires, transformers outside homes—and is highly segmented. Deviations from existing capacity can cause congestion and strain local equipment. The large demands from fleet charging almost certainly risk exceeding the local capacity. For example, Consolidated Edison, the electric utility in New York City, maps capacity at a fine geographic scale, demonstrating available capacity for EV charging at each transformer on the system. https://www.coned.com/en/business-partners/hosting-capacity Most locations throughout the city appear to be constrained to less than 200kW. Any significant new load, much less the huge demands required by large trucks and buses, would thus require upgrades to the local transformer and potentially other pieces of equipment before most depots could install charging stations.
This creates two challenges. First, local distribution investments are expensive. Bourlag, et al. (2021) estimate that the cost of installing or upgrading a feeder circuit of 5+ MW (only a portion of the upgrades needed) could be as high as $12 million (see Table 1).
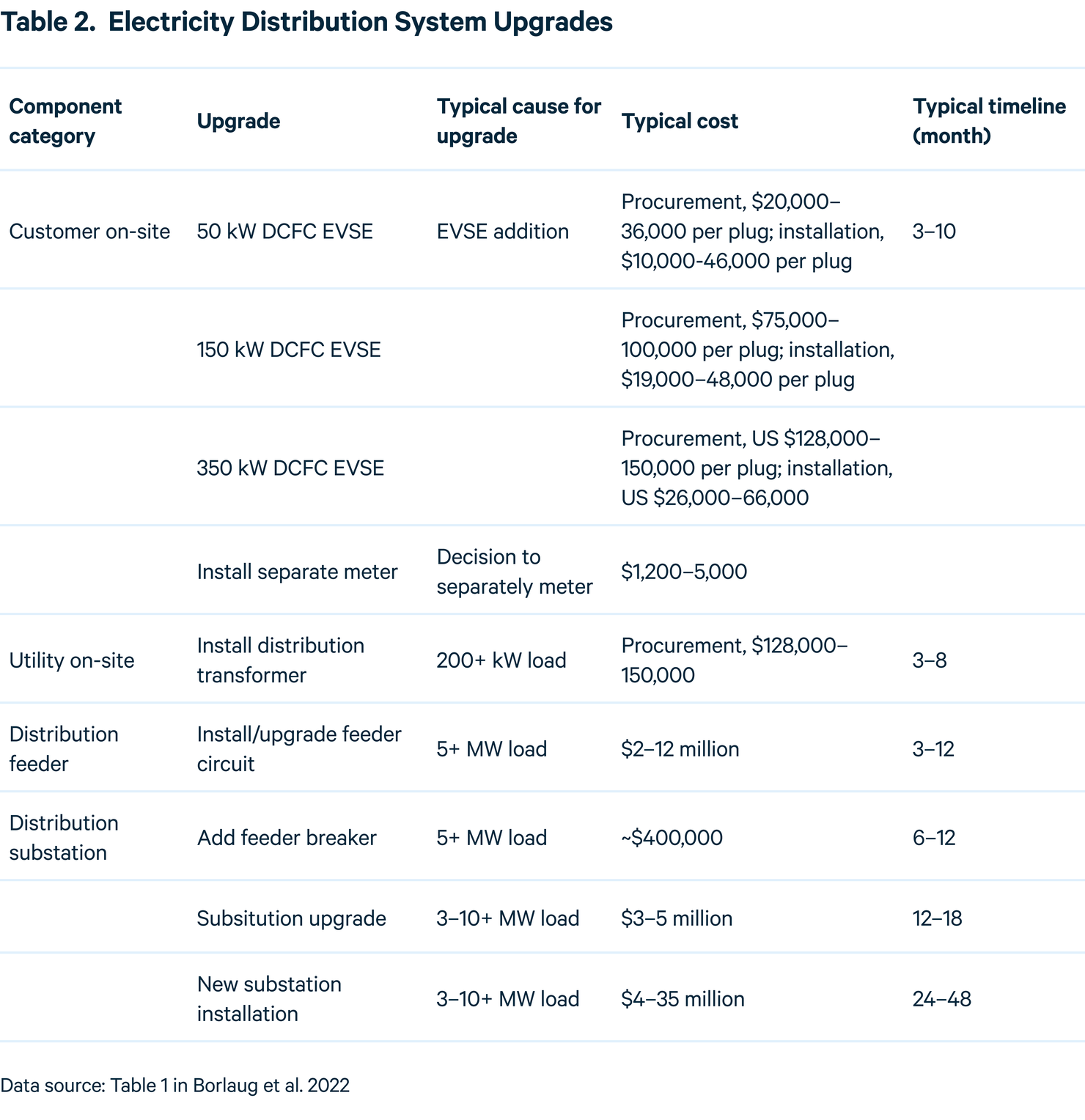
These investments contribute to retail electricity costs; thus, each fleet that is electrified will increase electricity costs to all ratepayers.
Second, making local upgrades takes time (Al-Hanahi et al. 2021) (Bourlag et al. 2022)—typically much longer than the time it takes to purchase an electric truck. If fleets are unaware of the timing discrepancy, they may purchase electric trucks and buses that cannot be charged at their depots.
5.2. Grid Reliability and Transmission
Ensuring that the grid is reliable is a core objective of electric utilities. Given the social and economic costs caused by blackouts and brownouts, utilities in many states have a statutory requirement to provide consistent power. Demand from large MHDEV fleets can destabilize the system and reduce reliability if upgrades are not done first. For example, Helou, et al. (2022) found that in Texas, simultaneous charging of even a few dozen vehicles could cause distribution-level voltage violations, and voltage collapse could occur if 38,000 vehicles are charged simultaneously; electrification of approximately 11 percent of trucks and buses could destabilize the transmission grid. Notably, the authors say that in real-world settings, protection equipment would prevent these vehicles from charging before any system collapse.
5.3. Generation Expansion Needs
To serve the electricity demand of MHDEVs, generation capacity will need to expand. Tong, et al. (2021) found that the electricity needed to charge long-haul vehicles in the United States amounts to 5 percent of total current electricity generation; this percentage will vary across locations. How much new generation is needed depends on when vehicles are charged; if charging occurs when generators are underutilized, such as at night, the system could rely on existing generators to provide at least some of the electricity needed.
To maximize the environmental benefits of electrifying trucks and buses, any extra generation used for charging needs to come from renewable sources. The extent to which renewables can provide this excess generation is an open question and will vary with the existing generation mix. For example, Talebian, et al. (2018) find that existing hydroelectric power generation would be insufficient for charging MHDEVs. Investment in other renewables will be required to meet the MHDEV charging demands with clean electricity.
5.4. Charging Station Investments
The final piece of infrastructure is the charging station. A charging station has two cost factors: the “make-ready” portion, and the charger. Make-ready infrastructure includes all the behind-the-meter wiring that allows the charger to be plugged into the grid. This portion of the cost may be paid for by the utility, though programs to provide this funding vary across service territories.
Figure 3. Infrastructure Required for Charging Station Investment
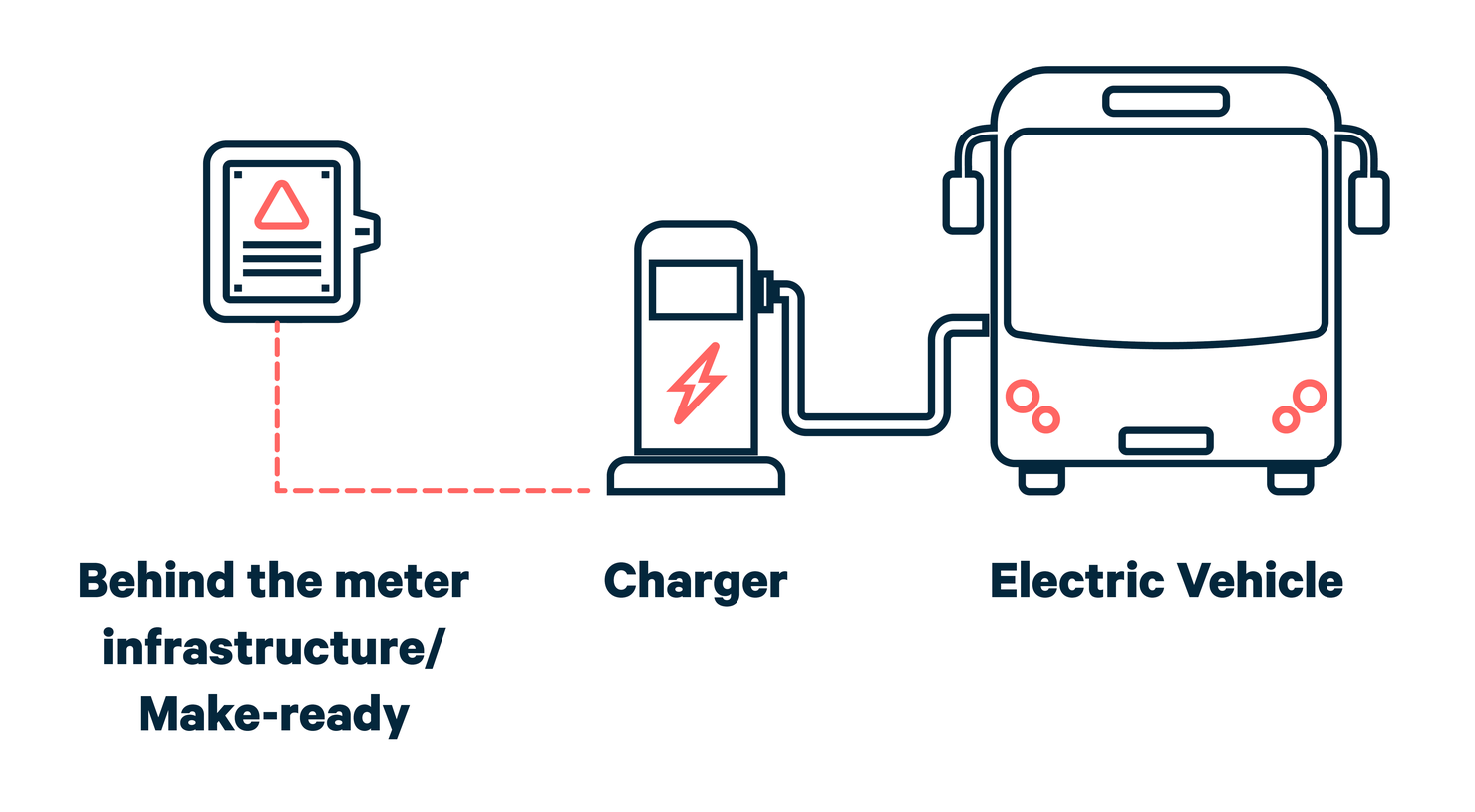
Fleets with depots will most likely purchase their own charging stations for recharging during their dwell periods. However, many other fleets will need to rely on public charging stations: those whose daily mileage exceeds the range of the battery, thus requiring midday, on-route fillups; fleets without depots, such as long-haul delivery trucks; and very small fleets and owner-operators, for whom the charging station investment is cost-prohibitive. Depending on location, the number of vehicles reliant on public charging may be large. For example, in California, two-thirds of all drayage trucks are expected to not charge at depot. https://subscriber.politicopro.com/article/eenews/2023/02/15/calif-clean-truck-mandate-may-kick-in-early-00082896?source=email Public charging stations set up for MHDEVs, however, are scarce today.
6. Challenge #5: Making the Transition Equitable
By addressing the transportation-related air pollution that disproportionately affects EJ communities, the transition to MHDEVs can reduce disparities and improve residents’ well-being and health (Camilleri, et al. 2022). The Biden administration’s Justice40 goals, which specify that 40 percent of clean energy investment benefits be targeted toward disadvantaged communities, facilitate an equitable transition to an electric transportation sector. An equitable transition does not mean just reducing pollution, however. With a thoughtful approach, the economic benefits of EV ownership and utilization can also be targeted toward these communities.
6.1. Ownership and Affordability
Owning electric trucks can benefit a company in several ways: these vehicles are cheaper, cleaner, safer, and more comfortable for drivers. Small fleets and fleets owned by racial or ethnic minorities, however, may be less able to access these vehicles, for several reasons.
First, small fleets often purchase used vehicles from large companies that sell their trucks after two or three years of use. The small fleets’ higher likelihood of buying used vehicles exacerbates inequalities across fleet size strata: larger, better-resourced fleets may hold on to their EVs longer, preventing the development of the secondary market that smaller fleets rely on. Second, because small fleets are more likely to purchase used vehicles, they may not be eligible for purchase incentives, which often focus on the new-vehicle market. Third, for small fleets that are expanding, having to scrap existing vehicles would compromise their goals.
Finally, low-income communities may not have the funds to purchase electric buses, garbage trucks, and other government-owned fleets, even though their residents may be most vulnerable to transportation pollution.
6.2. Ability to Access Incentives and Navigate Programs
Although many programs and tax credits now facilitate fleet adoption of MHDEVs, they entail bureaucratic and administrative hurdles, which may be particularly challenging for small fleets that lack financial experts. Some administrative hurdles can be overcome by restructuring the incentive—for example, by allowing the purchase incentive to be realized at the point of sale rather than requiring that it be claimed afterward.
Additionally, low-income communities that want to encourage MHDEVs may not have the tools to assess where charging stations should be sited to be most beneficial for residents. https://www.transportation.gov/rural/ev/toolkit/ev-infrastructure-planning/equity-considerations
6.3. Technical and Management Support for Financial Decisions
Fleets face many choices when deciding to electrify: Which models are best for the desired use cases? How many vehicles should the fleet buy, and when? Do routes and payloads need to be changed to accommodate restrictions on range and weight? Does the depot have enough electricity capacity to charge the fleet, and if not, when can the utility make these investments? A large fleet may be able to conduct analyses that would answer such questions and optimize TCO after purchase, but small fleets may not.
Grid capacity is a particularly challenging issue, and fleet operators may not know that utility investments will be needed up front. For example, a small fleet owner we spoke with had decided to electrify and had ordered electric trucks without realizing that his depot lacked sufficient power for charging infrastructure. The local electric utility informed him that the required upgrades would take a couple of years. He had to reroute power from other sources in the depot to the charging station, and even then, not all the vehicles could be charged.
Managed charging and co-located solar and storage (see Section 9.2) could help fleets reduce their electricity bills. Yet many small fleets, fleets owned by low-income and ethnic minority truck drivers, and fleets in EJ communities may not have the knowledge, connections, or credit to make these money-saving investments. Without these technologies, particularly managed charging software, they will be less likely to smooth their loads to reduce demand charges, and thus may face higher bills.
Merely having experience in dealing with the complex tariffs that come with high electricity demand can help reduce problematic charging patterns. For example, a school bus fleet may have only a small booth with a light in its parking lot and therefore have a straightforward, low tariff. Shifting to a complex tariff with demand charges and high rates can be a challenge. Again, whereas large fleets who have experience facing complex tariffs have dedicated energy managers and finance teams, small fleets are less likely to have this experience or these staff, and thus will face greater difficulty in managing their electricity bills.
7. Challenge #6: Externalities of the Transition
Externalities arise when actions taken by an individual or a firm impose costs (or deliver benefits) for others. When these externalities are unpriced, market outcomes may not be efficient from a general welfare perspective. In this section, we discuss some of these externalities and market failures.
7.1. Externalities for Sales and Use
Because they emit air pollutants, including nitrogen oxides (NOx), carbon monoxide, and particulate matter as well as greenhouse gases like carbon dioxide, diesel vehicles have significant negative externalities on public health and the environment. MHDEVs have several positive externalities. In addition to improving local air quality and reducing greenhouse gas emissions, they reduce dependence on imported oil, thereby improving energy security and reducing vulnerability to price fluctuations in the global oil market. They help support ancillary industries, such as charging infrastructure and more advanced battery technology. They are also quieter than diesel vehicles. When fleets make purchase and utilization decisions, however, they do not internalize the negative externalities of diesel vehicles (or the positive externalities of MHDEVs). This leads to an overinvestment in diesel fleets and an underinvestment in MHDEVs relative to the social optimum.
Moreover, depending on the electricity rate structure, fleets may not internalize the time-variant cost of electricity while recharging. Common across many utilities, a fleet faces a per kWh charge and a demand charge, neither of which varies over time. Its electricity bill depends on the total amount of energy used during the billing cycle and the maximum energy consumed during that period but is not affected by the timing of the consumption. Yet costs (both economic and environmental) vary by hour. Thus, the true hourly costs are not passed through to the fleet owner, leading to an overconsumption of energy during high-cost hours relative to the social optimum.
7.2. R&D Externalities
Technological improvements such as reducing energy density and optimizing vehicle design for greater battery range are crucial to scaling up MHDEV adoption, as are infant technologies such as battery swapping. However, a manufacturer cannot internalize all the benefits from its R&D investments, since competitors may imitate or improve on the innovation and capture the benefits. Because the manufacturer’s private returns from R&D are smaller than the social returns, it may underinvest in R&D. Similarly, MHDEV manufacturers do not fully internalize the benefits—improved air quality, lower greenhouse gas emissions, energy security—that result from their investment in these technologies, leading to an underinvestment in MHDEV manufacturing relative to the social optimum.
7.3. Environmental Externalities of Energy Supply
The emissions savings of MHDEV adoption will largely be determined by the source of the electricity supply. If the electricity used to charge the vehicles is generated from fossil fuels, the benefits of MHDEVs are attenuated. Therefore, transportation electrification must be complemented by expansion in renewable energy capacity, such as wind, solar, and hydropower. However, energy suppliers do not always internalize the entire climate benefits of supplying renewable energy and may therefore undersupply clean energy.
8. Alternatives to Electrification
MHDEVs may work well for certain medium- and heavy-duty applications, but high cost, low range and other challenges mean they are not yet viable for every use. Other low-carbon technologies that have developed alongside MHDEVs—hydrogen-powered fuel cell vehicles, biodiesel, and hybrid trucks—could fill these gaps.
8.1. Hydrogen Fuel Cell Electric Vehicles
Fuel cell electric vehicles (FCEVs) are expected to become cost-competitive with their diesel counterparts as early as 2035 (Ledna, et al. 2022). Recent literature has pointed to MHDEVs as a more economically viable solution than fuel cells (Ribberink, et al. 2021, Burke et al. 2022), but some long-haul applications could be better suited to hydrogen fuel cell technology, for several reasons (Brown et al. 2020). For instance, FCEVs have a much faster refueling time than MHDEVs (Brown et al. 2020). They also weigh less than MHDEVs, and hydrogen could be stored onboard where there is extra space, diminishing concerns about reduced payloads (Kast, et al. 2017).
Hydrogen comes with several drawbacks, however. First, today’s limited supply of hydrogen would need to increase for FCEVs to be economically viable (Brown et al. 2020). The source of the hydrogen is a second consideration. Hydrogen is primarily made with natural gas and thus could contribute to carbon emissions, negating the point of switching to alternative fuels (Cabukoglu, et al. 2019). For FCEVs to provide emissions savings, hydrogen fuel must be created with renewable energy, but that incurs an opportunity cost because it reduces the amount of green generation for powering the grid. Third, hydrogen is highly flammable and poses a safety concern: its storage and transportation would have to be handled with care to prevent endangering the truck driver and those who use hydrogen refueling stations (Anselma and Belingardi 2022, Otto, et al. 2022).
8.2. Biodiesel
Using biodiesel—whether 100 percent or blended with other fuels—could reduce greenhouse gas emissions by up to 74 percent compared with petroleum diesel (Huo, et al. 2009, Chen, et al. 2018). However, biodiesel production could affect land use (Delucchi 2010): increased production can require expansion of cropland and crowd out other land uses (Chen, et al. 2020). Biodiesel also incurs an opportunity cost of land for carbon sequestration: agricultural land for biodiesel production can’t be used to grow forests and store large amounts of carbon (Fehrenbach, Bürck and Wehrle 2023). Used cooking oil has been proposed as an alternative biodiesel that could avoid any potential land-use changes (Xu, et al. 2022).
Though biodiesel reduces carbon emissions, the air quality benefits are less certain. O’Malley & Searle (2021) suggest that biodiesel might harm human health by increasing emissions of nitrogen oxides. However, in 2021, the California Air Resources Board found that 100 percent biodiesel reduced NOx emissions by 5.4 percent relative to a diesel reference fuel (Durbin, et al. 2021).
8.3. Hybrid Trucks
Compared with their diesel counterparts, hybrid trucks have lower greenhouse gas emissions. In one study, average NOx reductions were 65 percent for hybrid diesel-electric vehicles (McCaffery, et al. 2021). However, the cost-effectiveness and environmental benefits of these vehicles will highly depend on the vehicle class and use case. For example, hybrids are most effective in uses where the driver starts and stops the vehicle frequently, allowing the benefits of regenerative braking to improve fuel efficiency (Dahodwala, et al. 2021, Stinson 2021); such benefits are not applicable to Class 8 long-haulers.
9. Policy Solutions to Maximize the Social, Environmental, and Economic Benefits of the Transition to MHDEVs
Well-shaped policies can help achieve two overarching goals. The first is to increase adoption of electric vehicles; to that end, the economics need to make sense from a fleet’s perspective. These vehicles are currently expensive to purchase, but policies can be crafted to help keep both upfront and operating costs down. Some policies can leverage the power of the market to reduce costs in an organic manner (such as creating electricity tariffs that incentivize managed charging to keep fueling costs low); others reduce prices without actually reducing the underlying costs (such as purchase incentives).
The second goal is to maximize the economic, environmental, and social benefits of electric vehicles after they have been adopted. For example, if these vehicles are charged by coal-fired electricity, the environmental benefits of adoption can dissipate. Similarly, if the electricity tariff is not carefully structured, the adoption of these vehicles can raise costs and reduce economic benefits of adoption—both for the fleet and for society at large.
In this section, we discuss policy solutions that can help achieve these simultaneous objectives:
- Lowering the upfront costs of adoption by, for example, reducing the cost to fleets of transitioning to these vehicles, reducing the cost of entry for manufacturers, and improving economies of scale.
- Managing operating costs and challenges through smart tariff design, requiring or incentivizing managed charging, investments in solar and storage, higher taxes on diesel fuel, and exemptions from weight regulations.
- Improving on-route charging options through smart and targeted public charging investments, battery swapping, and catenary charging (electric road systems).
- Reducing environmental and market externalities by greening the grid and allowing for more market spillovers within the manufacturing sector.
- Increasing adoption by small fleets through targeted subsidies and outreach and education.
Some policies are in place but can be adjusted to improve outcomes; others have not yet been implemented but hold promise for accelerating adoption and achieving more efficient outcomes.
9.1. Lowering Upfront Costs of Adoption
Transitioning to MHDEVs is costly. Policies can be leveraged to simultaneously alleviate high upfront costs in the short run while also targeting their root causes. Policy solutions can have short- or long-term benefits in making electrification more affordable.
9.1.1. Reducing Vehicle Purchase Costs
Market prices may decline naturally as the market develops. For example, thanks to improved technology, battery costs are falling. Burke, Sinha, and Fulton (2022) point out that the purchase price of MHDEVs may be competitive with that of diesel vehicles as early as 2025, though this is more likely to happen for smaller vehicles than for long-haul Class 8 tractors.
In the meantime, federal and state MHDEV subsidies can facilitate electrification by reducing private costs for the fleets. This is the case if the economic incidence of the subsidy falls on the buyer; under high market power, which may be the case in this setting (see Challenge #3), providing incentives to the purchaser may not be as effective in reducing the upfront purchase cost. The IRA of 2022 introduced a MHDEV purchase incentive of up to $40,000. The act also provides for $1 billion in grants for “clean heavy-duty vehicles” to support replacing Class 6 and Class 7 vehicles (buses, garbage trucks) with ZEVs, as well as the construction and operation of associated charging or fueling infrastructure. State policies, such as California’s Hybrid and Zero-Emission Truck and Bus Voucher Incentive Project and the New York Truck Voucher Incentive Program of the New York State Energy Research and Development Authority, provide vouchers for purchasing MHDEVs. See https://californiahvip.org/ and https://www.nyserda.ny.gov/All-Programs/Truck-Voucher-Program. In the short run, these subsidies only artificially reduce the price of adoption and do not address underlying costs. In the long run, they may affect the underlying costs by increasing adoption and improving economies of scale. The question remains, however, whether these incentives are big enough to accelerate adoption, and whether interactions with other policies can help reduce the burden on the government of subsidizing purchases.
Removing the excise tax on MHDEVs can also help reduce the purchase cost. Currently, Internal Revenue Code Section 4051 imposes a 12 percent federal excise tax on the first retail sale of a truck. Given that MHDEVs cost more, this excise tax increases the total tax burden for MHDEV purchases. To put it in perspective, the IRA’s $40,000 purchase incentive would cover only the excise tax on some very large trucks. An exemption on federal excise taxes for MHDEVs can thus help reduce the price differential with diesel vehicles and make purchase incentives more effective.
Battery leasing can also reduce the purchase cost by decoupling the battery cost from the rest of the truck. For instance, Proterra, an electric transit bus manufacturer, offers 12-year battery leasing, enabling fleet operators to purchase an electric bus for a similar price as a standard internal-combustion bus and lease the batteries over the 12-year lifetime. https://www.proterra.com/blog/financing-ev-fleets-with-proterra-battery-leasing-program/
9.1.2. Investing in Charging Stations
Policies can help reduce the private cost to the fleet of installing charging infrastructure. Given the importance of fast charging for commercial applications, support for Level III charging at home base locations may be crucial (Forrest, et al. 2020). At the federal level, IRS Section 30C provides credits for fleets to install charging infrastructure at their facilities. The IRA increased the previously allowable maximum tax credit from $30,000 to $100,000 for projects completed after December 31, 2022, with no limit on how many chargers a fleet can purchase; this can help fleets commit to larger investments in MHDEVs. At the state level, the EnergIIZE program of the California Energy Commission provides incentives for 50 to 75 percent of equipment costs for ZEVs, with a per project cap of $500,000 to $2 million. https://www.energiize.org/
Several utilities have implemented programs to cover the cost of the make-ready infrastructure. Rather than subsidizing the cost through government investments, these programs cross-subsidize through increases in electric tariffs across the utility’s customer base (Kresge and Steimer 2023). For example, the Consolidated Edison (ConEd) service territory has a Make Ready Pilot for MHDEVs, which provides up to 90 percent of utility-side infrastructure costs associated with installing the charger. Similarly, in PG&E’s EV Fleet Program, the utility covers the cost of all the electrical infrastructure from the transformer to the customer’s meter.
However, the participation requirements, the level of coverage, and fleet participation vary across programs. For example, whereas PG&E has an established program to cover these costs, ConEd has only a pilot, with limited funds and significant requirements for participation. The pilot funds are targeted toward EJ communities and are available on a first-come, first-served basis, with applications accepted only through 2025. Furthermore, to participate, fleets must also enroll in one of two programs (the New York Truck Incentive Program or the New York City Clean Truck Voucher Program), both of which help defray the cost of a new EV but require a diesel vehicle to be scrapped.
The scrappage requirement can dissuade fleets from participating, for two reasons. First, many fleets rely on selling their old vehicles to finance new-vehicle purchases. Scrapping the vehicle eliminates its resale value, thereby making the MHDEV investment more costly. Second, particularly in the short run, some fleets may hesitate to get rid of their diesel vehicles if they view the electric option as a risk; they may retain the diesel vehicles as backups. Scrappage requirements thus discourage participation in programs designed to reduce make-ready costs.
Furthermore, ConEd’s pilot covers not the full cost but only the least-cost make-ready. For example, it would fund the wiring of the charger to the street in front of the depot, but if the garage where the vehicles are parked is behind the depot building, the fleet would have to pay to bring the wires to the garage. In 2022, ConEd received no applications from fleets for the Make Ready pilot. In contrast, the PG&E program, which has no such cost-sharing or vehicle scrappage requirement, in 2021 had either signed contracts or activated sites for 116 charging locations, supporting the electrification of up to 1,800 MHDEVs.
9.1.3. Lowering Cost of Entry
Lowering the cost to enter and diversify products can encourage competition, thus limiting manufacturers’ market power and reducing the upfront purchase price of the vehicles. Incentives for manufacturers to add zero-emission offerings are one approach, as exemplified by ZEV credits in CAFE emissions standards, the EV manufacturing tax credit (26 U.S. Code § 48C This provides a 30 percent credit for any qualified advanced energy project, including MHDV manufacturing. Created in 2009, it was extended by the Inflation Reduction Act with up to $10 billion in funding.), and the Advanced Technology Vehicles Manufacturing Loan Program. This program has $17.7 billion in funding to advance electric vehicle manufacturing. The Infrastructure Investment and Jobs Act made these loans available to MHDV manufacturers.
R&D subsidies can also lower the cost of entry. An example of an R&D subsidy funded largely by the South Coast Air Quality Management District (SCAQMD) SCAQMD provided almost $45 million; the remainder came from Volvo and partners. that lowered the cost of entry was the Volvo Lights Project. Implemented in 2020, this $91 million collaboration of Volvo, the SCAQMD, and 12 other organizations, piloted the deployment of electric trucks in California, along with investments in charging stations and co-located storage and solar. The experience helped advance Volvo’s own learnings about manufacturing these vehicles while providing public learnings, disseminated through a lessons-learned guidebook. Volvo Lights Guidebook
Another policy to encourage entry is the Advanced Clean Trucks regulation by the California Air Resources Board, which requires manufacturers to sell increasing numbers of zero-emission commercial vehicles. Sales mandates implemented alongside purchase subsidies discourage pre-subsidy price increases because higher sales prices would dampen demand; thus, these two policies jointly can help keep prices down and sales high.
9.1.4. Increasing Demand of MHDEVs
Spreading upfront costs to enter the market and diversify offerings over greater numbers of vehicles will help manufacturers keep vehicle prices down. ZEV fleet mandates such as Advanced Clean Fleets by the California Air Resources Board can be a useful policy to achieve this goal. Mandates also help increase economies of scale, lowering the costs within and across manufacturers. Paired with vehicle purchase subsidies, fleet mandates can help ensure that the economic incidence of the subsidy is retained by the buyers.
9.1.5. Public-Private Partnerships
Because MHDEVs represent an expensive nascent technology with potential for large economic, social, and environmental benefits, it may be appropriate for government to step in and assume some of the risk associated with the transition. Public-private partnerships have been used by the Department of Defense to purchase large quantities of a new product at a high price to spur greater economies of scale or absorb the risk associated with the technology. For MHDEVs, the National Electric Vehicle Infrastructure Formula Program (“NEVI Formula”) is a public-private partnership to reduce risk to the private sector. Established by the Infrastructure Investment and Jobs Act of 2021, it provides $5 billion in funding for public charging stations, which can be targeted for commercial and MHDEV use. Under this program, the Federal Highway Administration makes grants to states to invest in a charging station network. States can then fund up to 80 percent of private investments in charging stations, including the upfront cost of the charger and operation and maintenance of the station (for up to five years). The program allows the state to enter into a cost- and revenue-sharing agreement whereby the private investor shares the revenues of the electricity sales with the state; these shared revenues must then be used to support the rollout, operation, and maintenance of the charging stations.
9.2. Managing Operating Costs and Challenges
Lowering operating costs will make the transition to MHDEVs affordable, but any reductions must not increase social costs. As an extreme example, if an electric utility completely subsidized the cost of charging, the free charging would accelerate adoption but also likely inflate peak demand and give fleets no reason to respond to price signals. Operating costs can be managed in several ways:
- Electricity tariffs can signal fleets to reduce maximum demand (e.g., by charging slowly, using the full dwell time to charge) and peak hour consumption (by shifting charging to off-peak hours).
- A low-carbon fuel standard or vehicle-to-grid (V2G) contract can help fleets earn extra revenue.
- Automated managed charging software can help fleets automatically respond to time-variant pricing.
- Co-located solar and storage can reduce the amount of grid-supplied energy needed to serve the load.
- Making diesel fuel more expensive (e.g., through taxes or cap-and-trade programs) can level the difference in operating costs across vehicle types.
9.2.1. Restructuring Electricity Tariffs
If the electricity tariff reflects the true cost of load at a granular temporal level, fleets save money by avoiding charging when the system is truly constrained and tariffs are high. Tariffs that reflect the true, time-varying system costs will be important to smoothing peak demands and making charging more cost-effective for fleet owners (Borenstein 2016, Phadke et al. 2019).
The ultimate effect, however, will depend highly on the fleet type. Some trucks and buses return to their depots overnight, and if the low-cost times align, they would naturally benefit from time-of-use charges (including demand charges). Other fleets may not have the flexibility to shift charging based on utility price signals because of their fixed operational schedules. Garbage trucks that run at night, for example, would need to change their operations to take advantage of low overnight tariffs. The effect of electricity tariffs on operational schedules and electricity bills is still an open question.
9.2.2. Generating Extra Revenue for Fleets
With vehicle-to-grid technology, vehicles can inject excess energy back into the grid. This technology is in its early stages of development and use, but it could become a way to reduce operating costs (Al-Hanahi et al. 2021). Importantly, the underlying electricity tariff or the contractual payment for the vehicle’s battery to engage in grid services will determine the profitability of V2G. Whether the fleet will have enough excess capacity will depend on vehicle type. School buses, for example, have excess capacity during summer and holidays, but vehicles that need to recharge completely overnight may be able to allow their battery to fluctuate only marginally. The flexibility and terms of the underlying tariff or contract will thus determine the benefit of allowing a vehicle’s battery to participate in grid injections or be used for voltage regulation.
A low-carbon fuel standard is another way to provide revenue streams for fleets. As implemented in California, it requires a reduction in the carbon intensity of transportation fuels that are sold, supplied, or offered for sale through 2030. See California Air Resources Board. The California program provides credits to charging station owners for installing DC fast-charging infrastructure. Fleets can generate revenue by selling credits to offset electricity costs and infrastructure investments.
9.2.3. Adopting Managed Charging
Managed charging encourages fleets to reduce their maximum demand during peak hours and thus maintain the reliability of the grid. It can be effective in reducing the costs of utility upgrades. For example, Hamilton & Couch (California Heavy-Duty Fleet Electrification 2021) found that managing charging could reduce peak demand for one fleet by up to 2.7MW. Spread over multiple fleets, this reduction is significant, and the cost savings can be tremendous. In fact, a New York study estimated that the distribution upgrades required to electrify transportation across the state would cost up to $26.8 billion; managed charging could cut this cost in half (Venkataraman and Speckman 2022).
Some fleets will voluntarily adopt managed charging software, given the financial benefits of doing so—particularly when they face demand charges and complex time-of-use tariffs. Fleets that pay a flat electricity tariff, however, have no incentive to manage their demand, and some fleets may simply not be aware of the benefits of investing in this software. Requirements that fleets use managed charging software (e.g., as a condition for receiving subsidies) would help keep peak demand down without having to rely on pricing incentives like demand charges. Subsidizing the software, which can cost thousands of dollars, and outreach and education on its benefits can also encourage adoption. Finally, an underlying tariff that creates incentives for managed charging leverages price responsiveness to help keep fleets’ operational costs down.
9.2.4. Promoting Co-Located Solar and Storage
By installing on-site solar photovoltaic generation and battery energy storage systems, fleets can reduce operating costs and peak energy demand for power from the grid. Hamilton & Couch (California Heavy-Duty Fleet Electrification 2021) found that on-site solar and storage installations resulted in the lowest total cost of ownership from electrifying heavy-duty fleets in California. Though the state’s low-carbon fuel standard provided an extra benefit to the fleet in that study, without it, co-located solar and storage can still help control costs. Some fleets may not be aware of the benefits of such investments. Policies to reduce the hurdle of adoption include an online marketplace for trusted solar-and-storage vendors, educational campaigns, and online tools; these can be provided by utilities or government agencies. Because solar-and-storage investments can be costly, financial incentives for adoption can be useful, particularly for small fleets.
A solar array on a warehouse of approximately 25,000 square feet could, on average, generate 2.2MWh per year, or only 260 kW of capacity. For large truck and bus fleets, instantaneous demands can exceed 5MW. Thus, rooftop solar may be able to offset only a small portion of demand. For fleets in rural areas, a solar farm next to the depot could allow a much larger installation.
The actual benefit depends on local utility regulations and rules. In West Virginia, net metering is allowed only for rooftop solar investments under a certain size; for commercial customers, the capacity cannot exceed 500kW (if the local utility is large) or 50kW (if small). https://www.solarunitedneighbors.org/westvirginia/learn-the-issues-in-westvirginia/net-metering-in-westvirginia/ But in California, the regulatory agency recently approved new net metering rules that will increase the benefit from installing solar plus storage, compared with solar only. https://apnews.com/article/business-california-utilities-public-commission-climate-and-environment-a1ecfe3fd3f34e8e2e10366a03f26158 The benefits of different types of investment are likely to change as rules and regulations are updated in response to greater adoption of distributed energy resources.
9.2.5. Equalizing Fuel Costs across Vehicle Types
R&D support for manufacturers to boost the gravimetric energy density of battery cells can help reduce the operating cost for MHDEVs compared with diesel vehicles. Diesel taxes are another approach. Washington State’s new tax on carbon emissions has increased the cost of diesel by about 59 cents per gallon. https://www.washingtonpolicy.org/publications/detail/states-new-tax-on-co2-emissions-projected-to-add-46-cents-per-gallon-to-the-cost-of-gas A carbon tax, however, may have some limitations: it may be regressive across the income distribution (Poterba 1989, Hassett et al. 2009, Mathur and Morris 2014), and is likely politically difficult to implement.
9.2.6. Allowing Weight Regulation Exemptions
Permitting MHDEVs to exceed maximum vehicle weight regulations, like California’s Zero Emission Vehicle and Near-ZEV Weight Exemption, can help fleets circumvent the logistical issues that arise from the heavy weight of the battery. A federal law that allows natural gas vehicles and plug-in electric vehicles to exceed weight limits by 2,000 pounds could be extended to battery electric vehicles. https://afdc.energy.gov/laws/11682 Not having to adjust payloads would improve the logistics and facilitate a fleet’s transition to MHDEVs, but weight exemptions can increase wear and tear on roads and raise safety concerns about crashes involving heavier vehicles. Exemptions may also face challenges from railroad companies that compete for shipping with long-haul trucks. Thus, weight regulation exemptions may be politically difficult to implement.
9.3. Improving On-Route Charging Options
MHDEVs that cannot charge overnight at depot will need alternatives, and policymakers can explore several ways to meet this need.
9.3.1. Investing in Public Charging Stations
Vehicles owned by individual operators or not housed at depots will depend on public charging. One public station for MHDEVs was created by Daimler near its headquarters in Oregon. This green parking lot, called Electric Island, provides MW-level charging that allows delivery trucks to fully charge in 20 minutes. https://www.bv.com/projects/electric-island-providing-pathway-carbon-free-trucking A public station in the Port of Long Beach, opened in 2022, provides charging for drayage trucks. But a full network of stations will be needed. https://www.porttechnology.org/news/port-of-long-beach-unfolds-electric-trucks-charging-stations/
This is a chicken-and-egg challenge: public charging station investments are unlikely to be made by private investors because of the limited adoption of MHDEVs, and MHDEVs are unlikely to be adopted as long as public charging is limited. Policies to accelerate public investment in charging stations can address this challenge.
The NEVI funds provide investment for public stations and shift the risk of low revenues to the government. However, states can provide funds for operational assistance to the charging station investor only for the first five years after the charging station is built. This will limit the incentive for investors, particularly where MHDEV traffic is low or uncertain, since the returns after those first five years will remain risky.
Green loading zones are dedicated MHDEV parking spaces that include a charging station. This provides a double benefit to the fleet owner in cities where parking is a problem. In New York City, for example, UPS fleets negotiate multimillion-dollar settlement payments with the city to cover tickets for double parking because so few commercial spaces are available. In 2019, UPS paid $23 million for parking violations, and FedEx paid almost $10 million. Green loading zones would provide both parking and recharging. New York previously considered green parking, yet it was not adopted (Salama, et al. 2014). NYSERDA conducted a study on green loading zones back in 2014 (Salama et al 2014). Green loading zones would require strict enforcement to prevent parking by gasoline and diesel vehicles.
9.3.2. Standardized Battery Swapping
Battery swapping would enable the quick and efficient exchange of a depleted battery with a fully charged one, extending the range of MHDEVs and helping the fleet plan logistics (Yukun 2023, Zhao, Wang and Fulton 2017). The replacement batteries can be charged slowly and during off-peak hours, eliminating the need for fast charging and reducing demand on the grid (Vallera et al. 2021). Moreover, this technology would also avoid battery degradation from fast charging and thereby improve the residual cost of MHDEVs, allowing them to compete in the secondary market. China, whose electric truck market accounts for around 90 percent of global electric truck registrations in 2022, prioritizes battery swapping and is a good example of how this can be implemented.
However, infrastructure to keep batteries stored, cool, and charged is expensive, and electric truck batteries can be heterogeneous. Therefore, battery swapping between different vehicles presupposes standardized battery design (Moultak et al. 2017) https://spectrum.ieee.org/ev-battery-swapping-how-is-this-a-good-idea and, accordingly, a coordinated effort among manufacturers. https://spectrum.ieee.org/ev-battery-swapping-how-is-this-a-good-idea It also requires building and maintaining a network of battery swapping stations, which will require more critical minerals than otherwise. Furthermore, this solution is unlikely to work for the heaviest vehicles because of the weight of the battery. For example, each of a Class 8 tractor’s four to six battery packs may weigh up to 1,500 pounds. Given such weights, swapping stations will likely be infeasible for much of the heavy-duty sector.
9.3.3. Installing Catenary Charging (Electric Road Systems)
Electric road systems are another solution (Liimatainen et al. 2019). These systems comprise a network of overhead wires, like those used by electric trains, that connect to the vehicle and transfer electricity to the battery. Catenary charging would allow longer ranges and smaller battery sizes, which are important trade-offs for MHDEVs, especially long-haulers (Moultak et al. 2017). The main downside is infrastructure cost. Catenary charging would require a network of chargers, which might be more feasible for highly connected systems than for sparsely connected long-haul routes (Moultak et al. 2017, Bernard, et al. 2022).
9.4. Dealing with Externalities
In the transition to MHDEVs, the benefits and costs that are not internalized by fleets or manufacturers include air pollution benefits of removing diesel MHDVs from the road, social and economic costs associated with MHDEV charging, and spillover externalities from technological innovation. Policymakers can align the private costs and benefits of an activity with its social costs and benefits through taxes and subsidies, command-and-control regulations, or market-based cap-and-trade mechanisms. Some of these policies can align incentives such that the adoption of MHDEVs maximizes the net social benefits of the transition.
9.4.1. Aligning Private and Public Incentives for Fleets and Manufacturers
Subsidies for MHDEV purchases or taxes on diesel vehicles and diesel fuel can help fleets internalize the true costs and benefits across fuel type when making purchase decisions. Alternatively, MHDEV purchase requirements can help increase demand. California’s Advanced Clean Fleets requires fleets to adopt an increasing share of electric vehicles through 2045, for example, and its Innovative Clean Transit rule mandates public transit agencies to shift to electric buses by 2040. Time-variant pricing (particularly real-time pricing) can help fleets internalize the time-variant cost of electricity while charging their vehicles and encourage them to shift charging to periods of low electricity demand.
Similarly, manufacturers would internalize the environmental benefits (costs) of selling MHDEVs (diesel vehicles) if policymakers provide incentives, such as advanced manufacturing tax credits and emissions regulations (e.g., CAFE standards). California’s Advanced Clean Trucks rule mandates that 100 percent of new MHDV sales be electric by 2045, for example, and will increase the supply of these vehicles. Finally, R&D subsidies can help manufacturers internalize positive spillovers.
9.4.2. Ensuring Green Generation Capacity
The electricity that powers MHDEVs must come from clean, renewable energy to maximize the environmental benefits of the transition. Several policies can help usher in a clean electricity system simultaneously with a clean transportation system. In addition to carbon taxes and renewable portfolio standards, cap-and-trade programs (e.g., the Regional Greenhouse Gas Initiative; see Schmalensee & Stavins, 2019) have been found to reduce power sector emissions. Other programs, such as investment tax credits and production tax credits for renewables, can also help; the IRA has provided $40 billion for the expansion of renewables through both types of credits (Newburger 2022). Finally, incentivizing co-locating storage and solar (such as through subsidies or other policies) provides a financial benefit to the fleet owner and can help reduce the reliance on the grid and total emissions from charging. A state-level policy that creates this incentive is California’s Low Carbon Fuel Standard (LCFS), which provides credits to fleet owners from charging their vehicles with renewable energy; Hamilton & Couch (California Heavy-Duty Fleet Electrification 2021) found that the LCFS heavily contributed to reduced TCO for California heavy-duty fleets.
9.4.3. Subsidizing Battery R&D
Battery technology has advanced even as costs have fallen by more than 90 percent in the past decades. Further technological improvements—greater capacity, higher gravimetric density—could increase the range of electric vehicles, making the transition to electric attractive to more types of fleets (Liimatainen et al. 2019). Therefore, R&D support for battery effectiveness could ease range concerns while also reducing the need for major investments in on-route public charging.
Furthermore, to prevent a shortage of critical minerals from interrupting the supply chain, investments in battery composition and battery recycling could help reduce dependence on certain scarce minerals.
9.5. Increasing Adoption by Small Fleets and Fleets in EJ Communities
Small fleets represent the large majority of trucking companies. In fact, only 2.6 percent of fleets have more than 20 vehicles; 91.3 percent have fewer than six. https://resources.lytx.com/blog/trucking-statistics-and-facts-for-fleet-managers Small fleets must therefore be the focus of the transition to MHDEVs, but voluntary adoption will be a challenge because of the high costs of electrifying and small fleets’ typical lack of access to credit. Because many trucks and buses are housed in or travel through EJ communities, which have been disproportionately affected by transportation pollution, electrification would provide significant welfare and health benefits. Policies can address these distributional and equity challenges.
9.5.1. Targeting Subsidies to Small Fleets
Targeted subsidies can help increase adoption by small fleets and fleets in EJ communities. Purchase subsidies could be extended to cover used vehicles, which small fleets typically buy. Incentives for new and used vehicles could be targeted toward fleets that are part of small businesses (Hardman, et al. 2021).
Large fleets are more likely to adopt in the short run, given their financing capabilities; will any funding be left when a small fleet decides to electrify? Ensuring that support is available for small fleets could be achieved by capping the number of vehicles within a fleet that are eligible for a subsidy, such that large fleets do not use up all available funding (Hardman, et al. 2021).
9.5.2. Providing Outreach and Leveraging Community Groups
Direct technical assistance is another way to help small fleets. Having an expert in electrification on staff would help small fleets adopt MHDEVs with all relevant logistics in mind.
Outreach will also provide help in navigating complex tariffs and taking advantage of utility programs. Some utilities have increased their outreach in hopes of engaging fleets interested in electrifying. For example, PG&E told us that they engage in this type of outreach to small fleets to make the first steps in building capacity for MHDEV charging. Community groups and trusted community leaders could help distribute information about the benefits of electrification and how to do so in a low-cost manner. Community groups can also identify small fleets for local utilities’ outreach.
9.5.3. Subsidizing Managed Charging Software and Co-Located Solar and Storage
The high upfront cost of managed charging software and co-located solar and storage put these technologies out of reach for many small fleets. Targeted subsidies can help ensure that fleets have access to low-cost charging while also reducing grid impacts.
10. Open Questions
MHDEVs are a nascent technology. The many uncertainties about the transition and market can make it challenging to craft policies for efficient, effective, and equitable outcomes. Research at different stages of MHDEV adoption, both during the transition and once these vehicles have been more widely adopted, can help improve policymaking and guide adjustments as conditions change.
Through our research, we have identified many open questions. Some require more experience with MHDEVs and more data; others could be answered through simulations and modeling.
10.1. Vehicle Use and Ownership
These questions concern the total cost of ownership and the environmental effects of adoption and vehicle use; answers can change how we structure policies for fleet adoption.
How do purchase and charging station subsidies affect the total cost of ownership?
Ex-post analyses of the economic incidence of purchase subsidies and sales mandates for MHDEVs will be valuable, but are challenging to conduct in the short run, given the limited sales and emerging policies.
Some incentive programs, such as the New York Truck Voucher Incentive Program and Colorado’s ALT Fuels, require that a fleet scrap a qualifying diesel vehicle. For light-duty vehicles, Ankney & Leard (2022) find that purchase subsidies with a scrappage requirement can result in fewer EV sales, more scrappage, and less program spending compared with a program without such a requirement. Although scrappage requirements might limit the adoption of EVs in the short run or affect used-vehicle prices, with consequences for purchases, the effects on truck and bus fleets’ purchase decisions, the secondary market, and environmental benefits are unknown.
How should electricity tariffs be reformed?
Theoretically, reforming electricity tariffs could lead to better outcomes: reduced demand on the system, reduced grid congestion, environmental benefits, and lower electricity bills. However, identifying optimal tariffs is a challenge. Variation in underlying costs makes it hard to compare observed tariffs across utilities because these costs determine the prices in a utility’s tariff; thus, only within-utility (and revenue-neutral) tariff comparisons are appropriate. Furthermore, heterogeneity across fleet types suggests that one set of optimal tariffs may not work for all fleet types and use cases. In the short run, simulations are needed to identify the effect of different tariffs on charging patterns and associated outcomes; once more fleets have adopted MHDEVs, ex post analyses of traditional and MHDEV-targeted tariffs can help improve tariffs.
What is the depreciation rate for MHDEVs, and the residual value of used vehicles?
The depreciation rate and residual values of MHDEVs are essential components of the TCO because they determine the sale price in the used-vehicle market. These values are currently unknown and are likely to depend on battery depreciation and advances in battery replacement and battery swapping technologies. As large fleets transition to MHDEVs, the speed of vehicle turnover may change. How will this affect the secondary market and the availability of electric vehicles? How will a shift to electric vehicles affect the resell timeline and small fleets’ ability to purchase used MHDEVs?
Uncertainty not only creates risk for fleets in adopting, it also reduces the accuracy of models that estimate how policies affect the used-MHDEV market. This research question will need to be revisited when there are more used EVs in the market.
How much would co-located storage and solar reduce the TCO? What about vehicle-to-grid technology?
What amount of solar and storage would be needed to achieve economic benefits to the fleet and reduced grid investments? What would be the cost of these investments? How quickly would these investments be amortized? Are there locations or certain sizes of fleets for which this investment would be more beneficial? How do the underlying electricity tariffs and rules about injections to the grid affect the decision to invest, the resulting charging patterns, and emissions?
Similarly, V2G may be more or less beneficial to the fleet depending on the contract and payment that the utility provides for injections to the grid, and the utility’s rules and regulations are likely to be very place-specific. Whether V2G can help with issues such as voltage regulation will depend on the fleet’s characteristics, such as availability and timing of the exports. Understanding the benefits of V2G for the system can determine the optimal payments and contract structures. Researchers can provide broad guidance on best practices related to injection payments and contract structures and utility regulation to ensure maximum efficiency and cost-effectiveness of V2G.
How would higher diesel prices affect MHDEV adoption?
Increasing the cost of diesel would lower the TCO for electric vehicles, but what price increase would prompt fleets to make the switch, given all the other challenges they face in electrifying? Comparing TCOs to determine the price point of the shift and raising the diesel price to the level at which the TCO evens out across fuel type is unlikely to have the intended effect because of the many transaction costs and other soft costs associated with adoption of MHDEVs. If other policies reduce the soft costs, the increase in diesel price may be higher or lower than what a TCO model would predict. Another question is whether customer-serving fleets can pass through fueling costs to their customers; that depends on the competitiveness in the market, customer loyalty, and the fleet’s market share. By exploring this question, researchers can help policymakers understand the distributional effects across fleet types of policies that raise diesel prices.
10.2. Manufacturing and Supply Chains
Answers to these questions can help identify optimal policies to accelerate the transition while ensuring that the market develops efficiently.
Would R&D investments lead to greater entry, product offerings, and innovation?
Given the low volume of MHDEV sales, the ability to conduct ex-post analyses of these policies has so far been limited. Conducting interim or preliminary analyses on the effect of the emerging policies and tax credits, particularly through modeling efforts to predict outcomes, can help policymakers refine their policy approaches in the meantime.
What is the value of technological spillovers in the MHDEV sector?
Emerging research on wind turbines suggests that initially, R&D produces greater benefits to the investing manufacturer, with limited spillovers to other manufacturers, but as demand grows, in aggregate these spillovers become meaningful and help reduce purchase costs (Covert and Sweeney 2022). Although the MHDEV sector, with its heterogeneous products and use cases, is very different from the wind turbine industry, the methodological approaches developed by Covert and Sweeney to study an emerging technology with limited cost information may be useful. The extent of spillovers both within and across manufacturers may help demonstrate the effectiveness of R&D investments and subsidies. For example, substantial cross-manufacturer spillovers may lead to suboptimal private investment in R&D, which rationalizes giving R&D subsidies.
How does market power affect vehicle prices?
With high product heterogeneity and high entry costs, market power may be more important than manufacturing costs in affecting prices. Understanding the extent to which this accounts for high prices is important for identifying policies to reduce purchase costs and for estimating the economic incidence of vehicle subsidy policies.
How are supplies and costs of critical minerals constraining development of MHDEVs?
How will the IRA’s restrictions Though these restrictions are binding only for light-duty EV subsidies, their stringency will affect critical mineral pricing and investments in mining, processing, and manufacturing, which will lead to changes in prices for MHDEV batteries as well. on critical mineral imports from non-free-trade countries affect critical mineral prices, and thus battery prices and demand for MHDEVs? How would technological innovation in battery mineral composition affect battery costs? What does battery swapping imply for critical minerals requirements, and can it lead to greater innovation in battery technology? Answers here can help identify effective policies to encourage battery innovation.
10.3. Public Charging Stations
Expanding the network of charging stations raises many questions.
How do public charging investments affect adoption decisions and interact with other incentives?
How would public charging investments change the logistics of adopting MHDEVs? What level of public charging station investment will promote electrification of smaller fleets and fleets not housed at depots? What is the cost-effectiveness of investing in public charging stations versus subsidizing MHDEV purchases or depot charging stations, and how do these two policies interact? With funds now available for private and public charging stations and MHDEV purchase subsidies, ex post analyses will soon be feasible.
What are the network issues for these investments and the charging market?
Where should charging stations be sited? Would certain locations across the country and in cities increase adoption of electric vehicles? What is the optimal pricing for public charging, and how are these prices regulated? What does the business model for these public investments look like? Finally, what regulations can help expand the charging station network?
How can public charging investments maximize benefits to EJ communities?
Certain corridors have disproportionately high truck and bus traffic, and low-income communities and communities of color are disproportionately exposed to the resulting pollution. https://cleanenergy.org/blog/equity-in-et/ How might public charging stations be sited to help reduce emissions in these neighborhoods? Would targeting particular transit corridors be more effective? Communities may oppose such investments if they increase truck traffic or reduce parking for passenger vehicles. A procedural justice question arises: who determines where these investments are made? Engaging with community groups will help ensure that these investments benefit the community, and identifying network and spillover effects in siting new charging stations—particularly in and around EJ communities—will be important for making efficient investments that reduce emissions.
11. Background
MHDEVs are a promising solution to decarbonizing the transportation sector. The technology is currently available for many use types and vehicle classes, and many vehicles on the market have a range that allows for normal operation in a fleet. Momentum for their adoption is coming from the federal government, which has signaled its interest in accelerating MHDEVs through the recent legislation; state governments that have adopted goals of achieving 100 percent new MHDEV sales; manufacturers, both traditional ones now making electric versions and start-ups that make only electric vehicles; utilities, which are taking steps to accommodate these vehicles’ demands on the grid; and communities that are demanding cleaner air.
Yet adoption of MHDEVs at scale is not an easy task. The shift to electric trucks and buses poses numerous challenges to the fleet owner, the electric utility, the manufacturers, and society. The transition will require policy coordination, investments, and action by multiple actors and stakeholders to keep costs down and maximize the benefits.
In this paper, we detailed many challenges associated with MHDEV adoption. First is how to make the economics of the transition work for the fleet such that the vehicles’ total cost of ownership—upfront cost of the vehicle and charging station, operating costs, and the vehicle’s residual value—is competitive with that of new diesel vehicles, in order to support the economic feasibility of transitioning to electric.
The second challenge is the fleet’s logistical issues: the vehicle’s range, the time it takes to recharge, the allowable payload, and the maintenance of the vehicle and charging station infrastructure.
The third challenge is manufacturing. The low demand for MHDEVs, idiosyncratic manufacturing process, evolving battery technology, heterogeneity across use cases, and supply chain restrictions deter manufacturers from entering the market, can give a few manufacturers high market power, thus maintaining vehicle prices high.
The fourth challenge is updating grid infrastructure to achieve electrification. MHDEVs’ large batteries necessitate cost-effective investments in the grid, transmission, and generation.
The fifth challenge is making the transition equitable. Electrifying transportation can improve air quality in disadvantaged communities. The fleets in such communities must be able to switch to MHDEVs affordably, so policies to accelerate MHDEV adoption must ensure that they can also benefit.
The final challenge is externalities, both market externalities (such as R&D spillovers and increasing economies of scale) and environmental externalities that can lead to underinvestment in MHDEVs, problematic charging patterns, increased emissions, and more.
All those challenges can be overcome with policies. Some policy solutions can even address multiple challenges. For example, structuring time-variant and cost-reflective electricity tariffs can help reduce the total cost of ownership by reducing charging costs; it can also motivate managed charging by aligning incentives, thereby reducing effects on the grid; and it would define the benefits of grid injections from electric vehicles. Similarly, R&D subsidies can increase the positive externalities of spillovers while also reducing the cost of entry into the market, thereby reducing prices and improving the total cost of ownership.
But there is much we do not know. If not properly structured, some policies could have unintended consequences. We therefore list open questions for further research—research that will be crucial for understanding the challenges associated with MHDV electrification and shaping policies to overcome them.