Carbon Pricing 301: Advanced Topics in Carbon Pricing in the Electricity Sector
How can carbon pricing lower emissions in the power sector? This explainer gives an in-depth outline of the central factors.
In Carbon Pricing 201, we explore how carbon pricing in the electricity sector reduces emissions and affects generation mix, dispatch, and retail electricity prices. Like economy-wide carbon pricing, carbon pricing can be implemented in the electricity sector through a cap-and-trade program or a tax on carbon emissions. This explainer explores how each of these policies can be implemented, design considerations for policy effectiveness and fairness, and lessons from existing regional programs.
Cap and Trade
Overview
A carbon cap-and-trade program for the electricity sector places a mass-based cap on the aggregate carbon emissions from emitting power plants. The cap on carbon emissions gradually declines over time to increase the stringency of the policy. Cap-and-trade programs target a particular level of emissions and are thus a direct approach to achieving specific emission reduction goals.
To comply with the policy, plants must possess an allowance (essentially, a permit) for every ton of carbon dioxide emitted. The number of allowances available in the market is equal to the policy’s cap, and they can be traded between plants. In theory, plants with a lower cost of abatement (emissions reduction) relative to the market price for allowances will choose to reduce emissions in order to sell allowances, while those with a higher cost of abatement will choose to procure allowances. Thus, a cap-and-trade program is economically efficient because emissions reductions across the sector will come from plants that can reduce their emissions at the lowest cost.
While the electricity sector is included in most existing cap-and-trade programs, some of those programs cover other sectors as well, including industrial plants and transportation. This is true of the California Cap-and-Trade Program, which covers power plants, industrial sources, and oil and gas distributors. The Regional Greenhouse Gas Initiative (RGGI), a regional cap-and-trade program consisting of ten northeastern states in the US (with Virginia joining in January of 2021), is the only program in the United States that exclusively caps emissions from the electricity sector. RGGI provides valuable lessons for designing a cap-and-trade program specifically for the power sector.
US Electricity Markets
In the United States, how electricity is bought and sold varies by region of the country. Electric utilities can be either regulated and operate as vertically integrated monopolies with oversight from state public utility commissions, or they can operate in deregulated markets where electric energy prices are set by the market with some federal oversight of wholesale market operations. These regulatory constructs determine how retail and wholesale electricity prices are set. To better understand the electricity market–related concepts discussed here in relation to carbon pricing, read “US Electricity Markets 101.”
Design Considerations
An electricity sector cap-and-trade program functions similarly to economy-wide cap-and-trade programs. However, there are a few key design elements that are particularly relevant for implementing such a policy for the power sector.
Allowance Allocation
The initial distribution of allowances to plants can be done either through an auction (where plants competitively bid for allowances) or through free distribution to certain plants (which can then trade them as they wish). Free distribution of allowances can be done in a number of different ways, but an important choice is whether the free allocation is based on a fixed measure, such as production or emissions at a plant for a specific year in the past, or on a changing metric, such as current or recent generation levels. The impacts of the policy on electricity prices for consumers, costs for producers, and society overall can vary depending on the allowance allocation method used and the regulation of electricity markets in the region. [Note: This explainer refers to several key concepts related to electricity markets. To learn more, please read “US Electricity Markets 101,” as the concepts are not necessarily defined or explained here.]
For power plants in a deregulated region with competitive wholesale power markets, a cap-and-trade policy with either auction allocation or free distribution based on a fixed measure leads to similar electricity price effects. The reason for this similarity is that in competitive markets, generators account for the value of allowances in calculating the offers that they bid into wholesale markets, regardless of how they obtained the allowance. Thus, even if they acquired the allowance for free, its value is factored into their bids. Otherwise, it would have made more economic sense to produce less electricity and sell the allowances rather than to use them to cover the emissions from producing electricity. The main difference between free distribution and an auction is that with free allowances, the value of the allowances accrues to generators in the form of potential windfall profits because a key input was obtained for free. With an auction, the value accrues to the government entity that runs the auction.
If allowances are allocated for free based on recent output, the effect on electricity prices is reduced. This approach to distributing allowances provides an incentive for generators to produce and sell more electricity to earn a greater share of allowances. As a consequence of this incentive, generators lower their bids in electricity markets to encourage more sales and electricity consumers see smaller electricity rate impacts.
By contrast, for power plants in rate-regulated jurisdictions with a cap-and-trade program in place, the value of emissions allowances is not included in the cost basis for plants’ average cost rates if the allowances are given for free because free allowances have a cost of zero to the utility. As a result, the price of allowances is included in the cost of service that forms the basis for average cost prices only if the allowances are auctioned. As a consequence, the price of electricity for consumers tends to increase more under an auction approach than under a free allocation approach.
Cost Containment
While cap-and-trade programs provide certainty for the quantity of emissions reductions, the cost of achieving those reductions can fluctuate because the price for allowances is determined through a market. Mechanisms can be built into an electricity-sector cap-and-trade program in order to prevent program costs from rising too high. One example is a cost containment reserve (CCR) that establishes a soft price ceiling for allowance prices in order to control program costs. If the market price for allowances rises above a certain threshold, the cost containment reserve is triggered, and a limited number of additional allowances is released into the market to keep the price from rising further. A cost containment reserve is a feature of both RGGI and the California Cap-and-Trade Program. Another mechanism, a hard price cap, sets a maximum price for allowances in a market, essentially translating the cap and trade market into a carbon tax at the cap price level. California is implementing a hard price cap starting in 2021.
Price-Responsive Allowance Supply
One drawback of cap-and-trade programs is that the cap serves as a ceiling for emissions reductions in that area. For instance, a sub-jurisdiction, such as a state or city, within a larger region that has a cap-and-trade program might attempt to impose additional measures to reduce emissions. This additional policy or program can reduce demand for allowances, freeing up allowances to be used elsewhere in the larger regulated region. As a result of this “waterbed effect,” one sub-jurisdiction’s efforts to reduce emissions has no effect on the overall emissions of the entire regulated region.
One example of this effect would be if one state within the RGGI region decided to take additional action to reduce emissions beyond the basic requirements of RGGI; for example, it might implement policies that support renewable energy or additional restrictions on emissions from generators within its borders. However, doing so would lead to decreased demand for allowances from generators within the state, and the extra allowances would become available for generators in other states within RGGI to purchase. As a result, these other states would be able to pollute more than they otherwise would have, thereby offsetting the benefits of one state reducing emissions through other policies.
One mechanism that helps to mitigate the waterbed effect is a price floor, also known as an auction reserve price, that prevents the allowance price from dropping below a certain price threshold and is a design feature in both RGGI and California’s Cap-and-Trade program. In RGGI and in CA, if the price floor is hit due to low demand for allowances, then the auctioneers withhold allowances from the market until the price rebounds above the floor. This feature can mitigate the waterbed effect by reducing the number of allowances in the market, which thus enables more ambitious emissions reductions from other policy measures when allowance prices are near the floor.
A natural extension of the price floor and another way to mitigate the waterbed effect and to promote emissions reductions from these additional policy measures is to implement a price-responsive supply of allowances whereby the level of the emissions cap depends on the market price of allowances. In RGGI, this type of price-responsive supply mechanism is called an emissions containment reserve (ECR). This mechanism ties the number of allowances available in the market (and thus, the amount of emissions permitted within the policy’s jurisdiction) to the allowance price. As demand and prices for allowances fall, a certain number of allowances would be withheld from the market. Burtraw and colleagues (2017) modeled the impacts of an ECR in RGGI and found that an ECR can be used to lessen the waterbed effect. Burtraw and colleagues (2020) consider what factors can affect the design of a price responsive supply curve that limits variability in allowance prices and allowance revenues with uncertainty about compliance costs.
The effects of the cost containment reserve, price floor, and emissions containment reserve are shown in the figure below. In Figure 1A, a drop in demand for allowances, perhaps resulting from sub-jurisdictional actions to reduce emissions beyond the cap, only lowers the cost of allowances while the emissions remain unchanged. However, as Figure 1B shows, the use of an Emissions Containment Reserve reduces the number of allowances in the market in response to a drop in price, thus enabling additional emissions reductions. The price floor has a similar effect on allowance supply but the quantity of allowances potentially removed from the market is not limited in advance. Instead the auction only removes any allowances necessary to keep the price at the price floor. Lastly, the cost containment reserve is shown in both figures and represents the price at which more allowances would be released into the market in order to contain costs.
Figure 1. Effects of Cost Containment Reserves, Price Floors, and Emissions Containment Reserves in Power Sector Cap and Trade
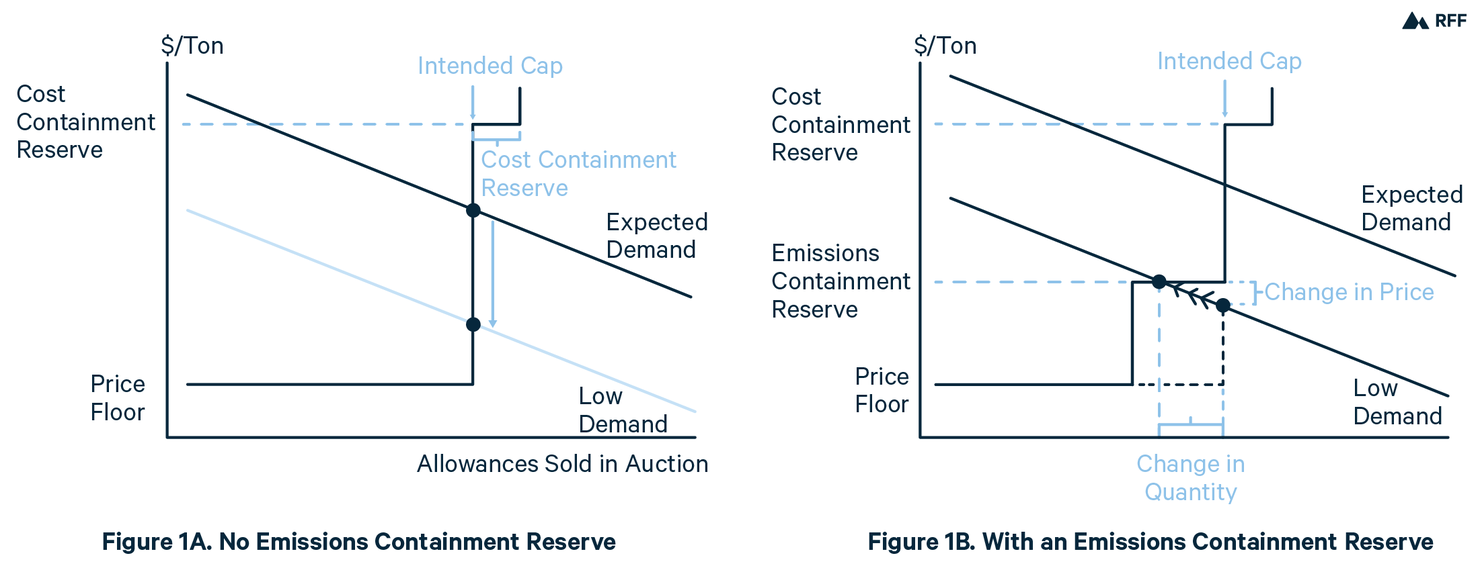
Leakage
Cap-and-trade policies can lead to increased emissions in regions not covered by the policy, which is called emissions leakage. Emissions leakage can reduce the effectiveness of a regional policy by offsetting some emissions reductions in the capped region with increases in emissions outside of the capped region.
A regional policy can increase wholesale electricity prices in the covered region relative to prices in other neighboring regions. As a result, the capped region may end up importing cheaper power from neighboring regions not subject to an emissions cap, and this power may be generated by carbon-intensive generating resources. Leakage occurs when capped and uncapped regions trade electricity or in wholesale markets where some of the plants are subject to a policy while others are not (e.g. if small generators are exempt from the cap).
RGGI, for example, has experienced some leakage to non-RGGI states. Since its creation in 2009, total carbon dioxide emissions from electricity generation in the RGGI region have dropped by over 35 percent. However, some of these reductions have been offset by emissions increases in non-RGGI states, particularly those that participate in the PJM regional wholesale electricity market. Because RGGI and non-RGGI generators in these states compete in the same wholesale power markets, those subject to the RGGI cap are more likely to have higher costs and operate at a competitive disadvantage, and therefore generators outside of RGGI are more likely to clear the market. In the case of RGGI, leakage to neighboring states Ohio and Pennsylvania resulted in a nearly 50 percent leakage rate, meaning that the policy led to an increase in emissions outside of the RGGI region that was equal to almost half as much of the emissions reductions that the policy achieved.
Design mechanisms can mitigate leakage to some extent. One solution is a border adjustment, which requires electricity imports to be subject to the cap. Another solution is a production incentive that distributes free allowances to polluting resources that are subject to a cap. The purpose of this method is to encourage these resources to bid lower in energy markets and thus be more competitive relative to imported electricity from polluting sources. Palmer et al. (2017) found that by providing existing natural gas generators with free allowances based on electricity production, 70 percent of emissions leakage could be avoided.
A third approach would be to expand the footprint of the cap and trade program, which would reduce the risk of leakage as more areas are covered by the cap. Such an expansion is already underway for RGGI; Virginia will join RGGI in 2021, and efforts are currently underway to develop a cap and trade program for generators in Pennsylvania that will link to the RGGI program.
Use of Revenue
A cap-and-trade program that uses an auction design raises revenue, and this revenue can be used to lessen the policy’s burden on lower-income households or improve the emissions performance of the policy. A cap-and-trade program increases electricity prices, which can have a disproportionate impact on lower-income households that spend a higher portion of their income on energy expenditures. Revenue raised from an auction, however, can be used to lessen the burden on lower-income households by offsetting electricity price effects or providing lump sum refunds to households (which is done in California with a portion of the allowance revenue). Revenue can also further the goals of the program if invested in clean technologies or energy efficiency measures, which can lead to lower costs and additional emissions reductions (possible with price-responsive supply) or to less leakage due to lower demand for imported power.
As of September 2020, RGGI auctions have raised over $3.7 billion since the program began in 2008. The use of these revenues for energy efficiency improvements, direct bill assistance, investment in renewables and selected other purposes has generated an estimated $4.7 billion in economic value for the RGGI states and has helped further reduce carbon emissions in the region (Hibbard et al, 2018).
Carbon Tax
Overview
A power sector carbon tax places a tax on emissions from the use of carbon-emitting fossil fuels for electricity generation. Unlike a cap-and-trade program, which provides certainty of emissions reductions at a market-determined allowance price, a carbon tax provides price stability but uncertainty regarding the quantity of actual emissions reductions.
Because a carbon tax sets an emissions price rather than a quantity, it can avoid some issues associated with cap-and-trade programs. For example, a carbon tax avoids the waterbed effect because all polluting entities are subject to the price, and therefore additional emissions reduction efforts by some entities will not affect the actions of others. However, like a cap-and-trade program, a carbon tax can lead to leakage, though similar solutions (like a border adjustment mechanism) are available for addressing it. The NY Independent System Operator (NYISO) has proposed a carbon tax for electricity generators within its region.
Design Considerations
Setting the Tax
Theoretically, an efficient carbon tax should be set to reflect the damages caused by each additional ton of carbon dioxide released into the atmosphere, more commonly referred to as the social cost of carbon. However, the social cost of carbon can be difficult to estimate and can vary depending on the method used. Additionally, in the United States, the social cost of carbon estimate is determined by a federal interagency working group, so the number has varied with the administration depending on the assumptions used.
Another method for setting the tax is to back-calculate a price that will achieve a certain target of emissions reductions. A few studies use this approach of calculating a price based on a specific emissions target (Kaufman et al 2020, Olsen et al 2018). The Olsen et al (2018) study in particular used specific information from ISO New England’s electrical system and fuel price data from the Energy Information Administration to determine the minimum tax rate needed to achieve a given level of emissions reductions and ran several sensitivities with varying assumptions regarding fuel mix. This nuanced approach can be useful for determining a tax rate for the power sector in a specific electricity market as it takes local constraints into account.
Increasing Emissions Reduction Certainty
Similar to the options available for flexibility in designing a cap-and-trade policy, a carbon tax can also be designed flexibly to accommodate changes in the market. For example, a tax adjustment mechanism is a design option for a carbon tax where the tax automatically adjusts up or down in response to changes in emissions reductions. If the policy is not achieving the intended level of reductions, then the carbon tax would automatically adjust upward to encourage more abatement.
Use of Revenue
A carbon tax on power sector emissions raises the price of electricity, and this price increase disproportionately impacts lower-income households since these households spend a larger fraction of their income on electricity than high-income households (Goulder et al., 2019). Whether the overall policy impact is regressive or progressive largely depends on how carbon revenues are used. Returning revenues through lump-sum dividends (either to all households or targeted to low-income households) is a progressive use of revenues and in some cases makes lower income households better off than they would be without the policy (the dividend payments are higher than their increase in costs). RFF’s Carbon Pricing Calculator shows how different proposed economy wide carbon taxes would affect people in different income groups. If revenue is recycled to assist lower-income households, the calculator shows that many households in lower quintiles can actually be better off with a carbon tax than without. While this tool is for economy-wide carbon pricing, effects of revenue targeting would likely be similar for an electricity-only policy.
For the electricity sector specifically, one option is to allocate the revenue back to utilities. To some extent, this method mitigates the effect of carbon pricing on consumers because it leads to smaller increases in electricity rates. This is the method that the New York Independent System Operator proposed for its electricity market carbon pricing proposal.
Other Considerations for a Power Sector–Only Policy
While a carbon pricing policy can significantly reduce carbon emissions from the power sector, efforts to decarbonize other sectors of the economy through electrification could be negatively impacted by a power sector–only policy. For example, if electric vehicles are subject to a carbon price through electricity usage but gasoline vehicles are not, the policy could discourage switching from gasoline to electric cars. If a power sector–only policy is implemented, this effect could be addressed through measures such as by exempting electric vehicles from the carbon tax or using the carbon tax revenue to promote the adoption of electric vehicles. However, on the whole, an economy-wide carbon price (which includes the power sector) would be more effective for addressing total emissions than a power sector–only policy.
Another unresolved issue is the role of the Federal Energy Regulatory Commission (FERC) in oversight of carbon pricing. To the extent the carbon pricing policies are administered by states, FERC has largely not played a role in direct oversight. As carbon policies get more ambitious and costs increase, the effects of carbon pricing on transactions in multi-state wholesale power markets that include states with and states without carbon pricing will pose challenges to those markets. These differences across states will also challenge the environmental efficacy of the state policies due to emissions leakage. In such situations, FERC may be called upon to address inconsistencies in state policies that impact its markets through imposition of border charges or other mechanisms. In addition, if regional grid operators decide to advance carbon pricing within their wholesale energy markets, as the New York Independent System Operator has proposed, then FERC will have some jurisdiction over these policies and oversight of their design.
Conclusion
Reducing carbon emissions from the power sector is critical for mitigating climate change. Pricing carbon through a tax or cap-and-trade program can efficiently encourage a transition to a cleaner electricity system by promoting the use of cleaner fuels and the conservation of energy.
Regional cap-and-trade programs have successfully reduced emissions from the US electricity sector, and these programs provide useful lessons for future policies. Some policy proposals, like New York’s proposed carbon price in wholesale energy markets, could also be useful for understanding how a power sector–focused carbon tax could work.
While regions have experimented with carbon prices, national carbon pricing has yet to materialize despite numerous proposals at the federal level. In the absence of a federal policy, another option for reducing carbon emissions from the electricity sector is a power-sector policy called a clean energy standard (CES), which is similar to a renewable portfolio standard (RPS) policy that more than half of US states currently use. A CES is a technology-neutral portfolio standard that requires a certain percentage of electricity sales to come from “clean”, or low or zero-carbon, resources, and its crediting system can mimic design features of carbon pricing without directly pricing carbon. More information on a CES can be found here.
Sources
- Burtraw, D., Palmer, K. L., Bharvirkar, R., & Paul, A. (2001). The effect of allowance allocation on the cost of carbon emission trading (No. 1318-2016-103258).
- Burtraw, D. Holt, C., Palmer, K. and Shobe, W. (2020). Quantities with Prices: Price-Responsive Allowance Supply in Environmental Markets, Resources for the Future working Paper
- Burtraw, D., Holt, C., Palmer, K., Paul, A., & Shobe, W. (2017). Expanding the Toolkit: The Potential Role for an Emissions Containment Reserve in RGGI. Report. Washington, DC: Resources for the Future.
- Fell, H., & Maniloff, P. (2018). Leakage in regional environmental policy: The case of the regional greenhouse gas initiative. Journal of Environmental Economics and Management, 87, 1-23.
- Goulder, L.H., Hafstead, M.A.C., Kim, G., and Long, X. (2019). Impacts of a carbon tax across US household income groups: What are the equity-efficiency trade-offs? Journal of Public Economics, 175, 44-64.
- Hibbard, P. J., Tierney, S. F., Darling, P. G., & Cullinan, S. (2018). An expanding carbon cap-and-trade regime? A decade of experience with RGGI charts a path forward. The Electricity Journal, 31(5), 1-8.
- Kaufman, N., Barron, A. R., Krawczyk, W., Marsters, P., & McJeon, H. (2020). A near-term to net zero alternative to the social cost of carbon for setting carbon prices. Nature Climate Change, 1-5.
- Olsen, D. J., Dvorkin, Y., Fernandez-Blanco, R., & Ortega-Vazquez, M. A. (2018). Optimal carbon taxes for emissions targets in the electricity sector. IEEE Transactions on Power Systems, 33(6), 5892-5901.
- Palmer, K., Burtraw, D., Paul, A., & Yin, H. (2017). Using production incentives to avoid emissions leakage. Energy Economics, 68, 45-56.
- Raimi, D., Keyes, A., & Kingdon, C. (2020). Florida Climate Outlook: Assessing Physical and Economic Impacts through 2040. RFF Report (20-01).
- Stavins, R. N. (2008). Addressing climate change with a comprehensive US cap-and-trade system. Oxford Review of Economic Policy, 298-321.