Federal Climate Policy 105: The Industrial Sector
An overview of federal policy options for reducing greenhouse gas emissions from the US industrial sector, from standards to funding for research and development.
Reducing industrial emissions of greenhouse gases (GHGs)—primarily carbon dioxide (CO2), but also some nitrous oxide and methane—has received little policy attention compared to the power and transportation sectors, in spite of industry’s large and growing carbon footprint. In 2019, the industrial sector produced 29.6 percent of all GHG emissions in the United States, when including emissions from generating electricity used by industry (Figure 1).
Figure 1: US Greenhouse Gas Emissions by Sector in 2019
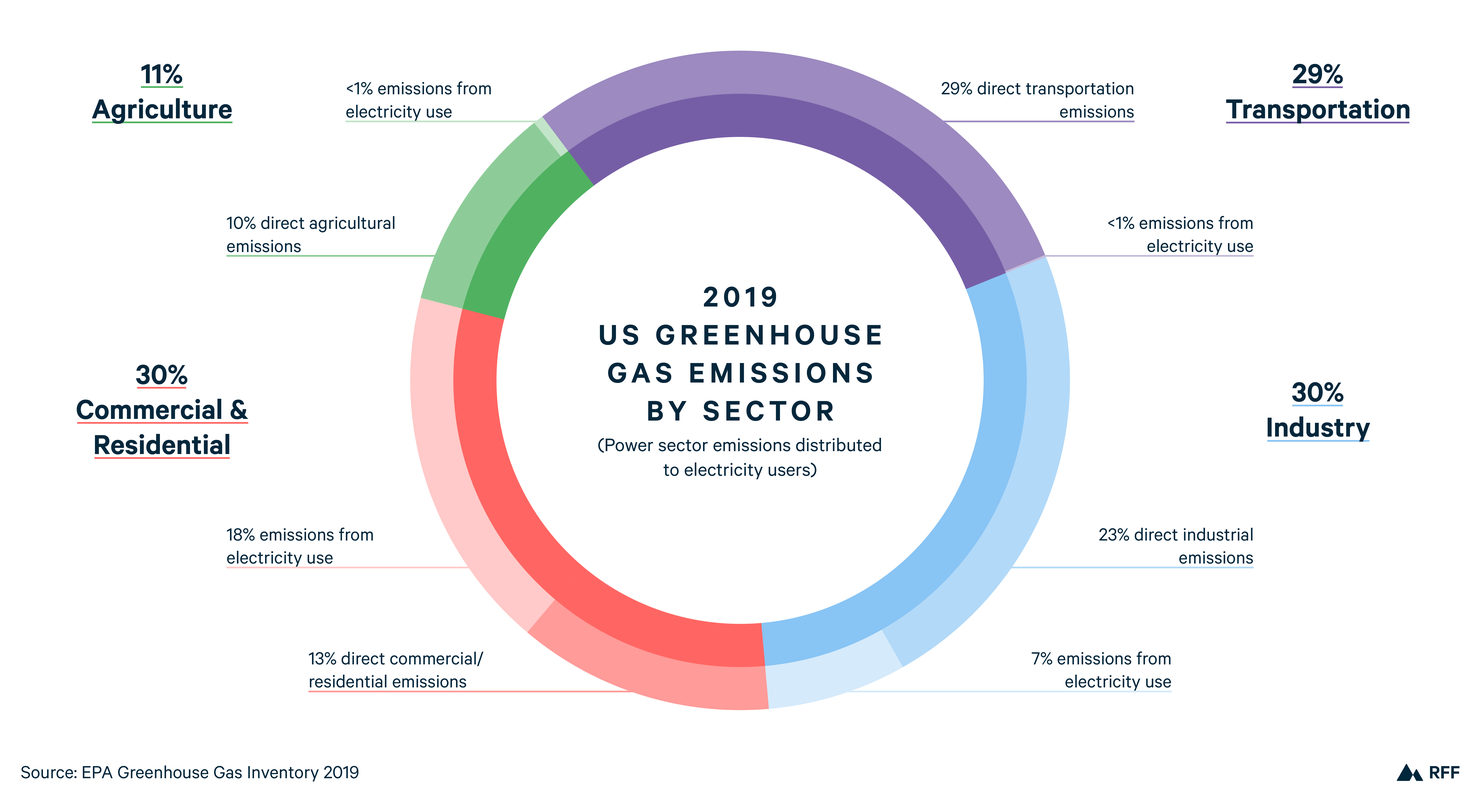
The majority of industrial emissions (78 percent) comes directly from industrial processes, heating, and other uses, while about 22 percent are emissions that arise indirectly from the use of electricity within the sector. The industrial sector includes a diverse set of activities, such as manufacturing goods and producing materials like steel and cement.
Sector Overview
The industrial sector encompasses a diverse set of products and processes with widely varying levels of emissions and emissions intensity (the amount of emissions relative to the dollar value or quantity of product produced). Figure 2 shows the emissions intensity and share of the manufacturing subsectors with the highest emissions intensity (data from Rhodium Group analysis).
Figure 2. 2018 GHG Emissions and Emissions Intensity of Manufacturing
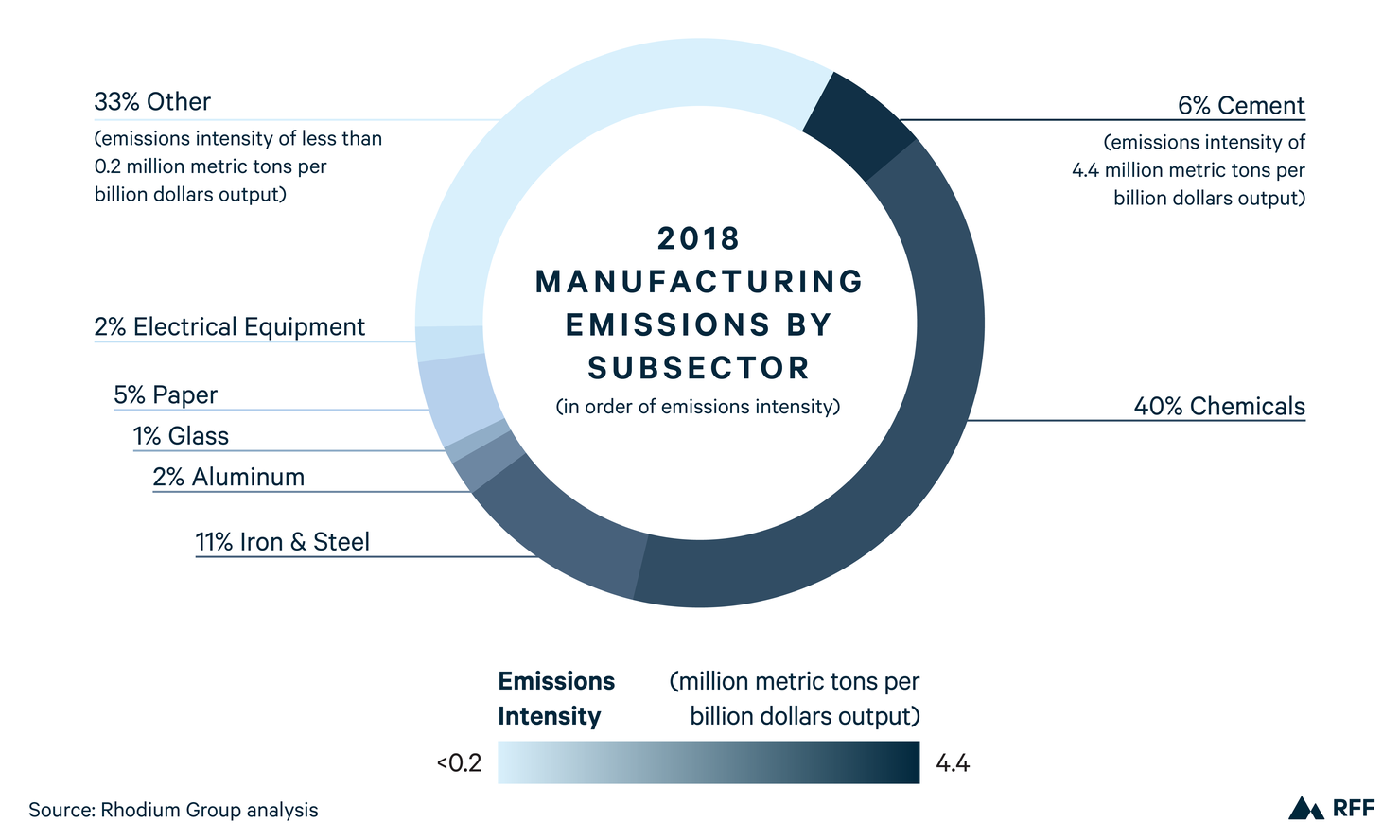
Given the characteristics of the industrial sector, several mitigation pathways are possible—and likely necessary—to reduce industrial emissions:
- Incentivizing a shift away from high-carbon energy sources and high-carbon manufacturing processes toward lower-carbon energy sources and processes (e.g., low-methane-emissions natural gas, decarbonized hydrogen, biogas, and electrification of production processes and heat sources).
- Encouraging industrial sources to capture the CO2 they generate
- Reducing energy use by increasing the energy efficiency of production processes and heat systems.
- Cutting material use and planning for the “circular economy” through product designs and manufacturing standards that reduce waste and one-time use.
- Decarbonizing the grid. Reducing the emissions associated with generating and transmitting electricity will lead to industrial emissions reductions, particularly if the industrial sector becomes more electrified. While this pathway would not directly involve the industrial sector and would increase electricity costs, no changes in industry investments, fuel use, or production processes would be necessary.
- Accelerating technological development that reduces costs or increases the efficacy of the other five pathways.
Policies can target one or more of these pathways—and some policies can operate on all six at once. For example, a carbon price applied to all industrial sources of CO2 would encourage factory managers and company owners to find the cheapest ways of reducing emissions and avoiding carbon fees, thereby indirectly stimulating innovation. However, sufficiently high carbon prices to decarbonize the industrial sector may be politically infeasible. Also, most emissions policies operating directly on the industrial sector cannot sufficiently incentivize innovation, so policies promoting research, development, and demonstration still would be needed (for more information about carbon pricing, please read “Federal Climate Policy 102”).
In addition to considering the mitigation pathways above, policymakers can think of industrial decarbonization in a few different ways:
- By subsector or product: Some policy strategies may work to decarbonize a specific subsector (such as refineries) or product—for instance, a piece of legislation may aim to reduce emissions from cement manufacturing specifically.
- By process: A policy might be implemented to decarbonize a process that is commonly used among multiple or single industrial subsectors; for instance, a standard to improve boiler or motor efficiency, or a limit on emissions from blast furnace steel production.
- By fuel: A policy might focus on a specific fuel; for instance, regulations may restrict the use of coal, or tax credits could encourage the use of certain low-carbon fuels.
A variety of policy approaches to reduce industrial emissions along one or more of these pathways exist. While these types of policy options may be less efficient than a carbon price, these alternatives nonetheless may be more politically feasible. This explainer examines several of these options.
The example of hydrogen is used in several places throughout this article as a major technological opportunity for industrial decarbonization. Other opportunities exist, including energy efficiency; electrification; fuel switching from coal to gas; carbon capture, utilization, and storage (CCUS); and various industry-specific technologies. The recent interest in hydrogen to dramatically decarbonize across many industrial sectors in the long term has distinguished it as a significant area of opportunity, and thus the focus of our examination. A 2020 RFF report explores the opportunity of decarbonized hydrogen in more depth, and an accompanying graphic illustrates different hydrogen production methods. Learn more on hydrogen tax credits in the info box below.
Hydrogen Tax Credits
Hydrogen can be used as a fuel. Emissions benefits for switching to hydrogen from other fuels depend on the energy sources used to generate both the hydrogen and the fuel that hydrogen replaces.
Hydrogen is produced in a variety of ways. Most hydrogen in the United States is made by natural gas reforming (gray hydrogen) which uses high-powered steam on a methane source (e.g., natural gas) to produce hydrogen, carbon monoxide, and a small amount of carbon dioxide. Gray hydrogen is carbon-intensive, consuming roughly 6 percent of the US natural gas supply. Emissions also are substantial, because carbon capture from the main hydrogen production processes—natural gas reforming (gray hydrogen) and coal gasification (brown hydrogen)—is rare. Currently, hydrogen production emits 830 million tons of CO2—more than 2 percent of global CO2 emissions, all of which could be eliminated at no direct cost to users.
Unfortunately, methods of decarbonizing hydrogen by adding CCUS to hydrogen production processes (creating blue hydrogen) or powering electrolysis with carbon-free renewable or nuclear electricity (to make green hydrogen) are currently too expensive to allow decarbonized hydrogen to compete in the market without policy incentives. Of all the policies available in the near term to support production, a hydrogen tax credit may be most viable. Such a credit would permit eligible producers and industrial users to claim a deduction on their taxes. By setting the credit at a suitably high level, policymakers could help blue hydrogen become competitive for refineries and ammonia manufacturers, which already make gray hydrogen for their production processes. A hydrogen tax credit could reflect the social cost of carbon; or match the established levels for 45Q, the CCUS tax credit program; or offer a larger credit to push decarbonized hydrogen even faster.
Tax Credits
The Basics
Tax credits enable emitters to pay lower taxes in exchange for reducing their emissions. Tax credits are flexible: they can be designed to reduce CO2 emissions broadly, or they can be more targeted. Under a broad tax credit policy, industrial emitters can decide how to reduce their emissions. Alternatively, a credit program can favor specific technologies; for example, investment and production tax credits exist for solar power, wind power, and CCUS. Economists typically consider the designation of technological “winners” as economically inefficient, but tax credits that target certain technologies can create incentives for innovation in the private sector that ultimately will reduce the costs of the favored technologies.
Benefits and Challenges
A tax credit—a subsidy by another name—is far more popular than pollution taxes among most policymakers and regulated entities. A tax credit has the advantages of being a familiar federal policy with a decent track record (e.g., in stimulating renewables).
However, like most policy options, tax credits have some drawbacks. They are often expensive, and they tend to be narrowly drawn, potentially excluding promising options by “picking winners” when the credits target certain technologies. They also may not be sufficient aloneon their own. For example, even with a tax credit, low-carbon hydrogen production may not be economical. The most efficient tax credit would be based on actual reductions in CO2 emissions, rather than the production volume or amount of money invested in renewables.
In spite of these drawbacks, among the policy tools that can help start the process of decarbonizing the industrial sector, tax credits have the advantages of practicality, familiarity, and industry support.
Key Considerations
Several factors are important to consider for an effective tax credit. The most critical are described below.
The basis for the credit and who it is assigned to: A key decision to be made in designing a tax credit is who receives the credit and what they must do to receive it. For example, with decarbonized hydrogen, producers can receive a credit if they reduce the emissions associated with hydrogen production; or users who purchase decarbonized hydrogen can get a credit for replacing high-carbon hydrogen, fuels, and industrial feedstocks with lower-carbon alternatives; or both producers and users can receive a credit through two different credit systems. The choice depends largely on the ease of accounting; for instance, it may be easier to assess whether a producer is meeting the tax credit eligibility requirements than an end-user, or vice versa.
Coordination with other tax credits: For any tax credit, it is necessary to review how it overlaps with other tax credits and avoid double-counting. For instance, the existing 45Q tax credit for carbon capture and storage could overlap with a tax credit for decarbonized hydrogen.
The level of the credit: The level of the tax credit—the amount of money awarded for the desired action—can be set in two basic ways: based on the social cost of carbon or based on the value that is necessary to motivate the desired action (such as switching from coal to natural gas as a fuel).
Additional considerations: Additional important factors to consider in designing a tax credit include defining who is eligible to receive it; determining the timeline (such as when production or investment must begin in order to qualify, and when credits are available); and requirements for monitoring, reporting, and verification.
Past, Current, and Proposed Tax Credits
As noted above, the tax credit is a potentially productive approach to encouraging emissions reductions and stimulating technological innovation. This policy mechanism has also been used in other sectors with success.
In the industrial sector, the 45Q tax credit for CCUS has led industrial entities to seek approval for their projects and apply for credits from the IRS.
Energy Efficiency Standards
The Basics
Improving energy efficiency is a proxy, albeit imperfect, for reducing CO2 emissions in the industrial sector. Energy-efficient technologies have promise for reducing the costs and environmental damages associated with energy use, but these technologies are not being leveraged to their full economic benefit by businesses or consumers. Policies that encourage the adoption of energy-efficient technologies can both benefit the industrial sector and reduce emissions. Note that the private energy-efficiency gap is generally smaller than the social energy-efficiency gap, which considers the broader societal damages associated with energy use.
Two types of energy efficiency standards are commonly discussed:
- Prescriptive standards (also known as technology standards) typically require a particular energy-saving technology or process to be installed or used. Historically in the environmental field, prescriptive standards have been applied most often for reducing pollutants—but they could be applied to the industrial sector, as well.
- Performance standards generally limit the emissions or energy consumption per unit of product (e.g., amount of GHGs emitted per ton of cement manufactured). Unlike prescriptive standards, performance standards do not specify the technology or design details of the process.
Benefits and Challenges
The principal advantage of energy efficiency standards is the reduced use of energy as well as generally lower GHG emissions (and conventional pollutant emissions). Lower energy use often reduces operating costs as well, which can offset some or all of the higher capital costs. The standards are also generally transparent, which simplifies enforcement.
The principal disadvantage of energy efficiency standards is the increased capital costs of the products or processes covered by the policy, or potential reductions in performance. Further, prescriptive standards do not allow much flexibility for producers and can lock in particular technologies, which can forestall technological innovations.
Key Considerations
When designing energy efficiency standards, policymakers should consider the causes of the energy-efficiency gap. As discussed in the literature, multiple factors can help the failure to adopt the full suite of economically attractive technologies, including firms’ market power, limited information about the new technologies, the presence of unobserved costs, a lack of understanding of a firm’s own energy operating costs, and firms’ inability to capture the full benefits from RD&D. Policies should be designed to address the specific causes of the energy efficiency gap.
Past, Current, and Proposed Energy Efficiency Standards
There are several energy efficiency standards in place at the federal level in the United States. In the transportation sector, for instance, Corporate Average Fuel Economy (CAFE) standards regulate the amount of fuel cars use to drive a given distance.
Energy efficiency standards are in place for appliances, which are relevant to the industrial sector and the buildings sector. The National Appliance Energy Conservation Act of 1987 established minimum efficiency standards for many common household appliances. The Energy Independence and Security Act of 2007 updated or enacted standards for 13 products.
Starting in the late 1990s, the US Environmental Protection Agency established ENERGY STAR and other industry-oriented, nonregulatory, and voluntary programs that certify products (e.g., cement) which help reduce GHG emissions by reducing the amount of energy consumed per unit product. Typically, products with such certification sell for a higher price per ton and can be eligible for green procurement programs.
In principle, one could build on these experiences, from CAFE standards to ENERGY STAR, to develop new process or product standards for industry CO2 reductions.
Tradable Performance Standard
The Basics
A performance standard is a policy that sets a benchmark that firms must meet, without specifying how the benchmark should be achieved (for example, a limit on the emissions that result from manufacturing each ton of cement). A tradable performance standard (TPS)—sometimes referred to as a “clean energy standard” for industry—is a flexible mechanism that encourages firms to use less carbon-intensive materials and employ production techniques that lead to lower emissions.
The “tradable” aspect of a TPS refers to the ability of firms to buy and sell credits with one another. This can improve overall cost-effectiveness and encourage innovation that in turn leads to lower emissions, as companies can make money from selling excess credits.
In principle, a TPS could be set for each industrial category or sub-category, requiring firms to meet emissions or other benchmarks on the basis of the quantity or dollar value of product sold. Depending on the design of the system, a TPS for industry might allow trading within each sector and/or across sectors. Certain elements of existing cap-and-trade programs are carried over to TPSs. A TPS can create incentives for firms to reduce emissions, similar to a cap-and-trade program that allocates emissions allowances in proportion to a facility’s output. Examples include California’s economy-wide cap-and-trade program and the European Union Emissions Trading System.
Benefits and Challenges
Compared to an energy efficiency standard, a TPS is more flexible and is almost always more cost-effective.
A major advantage of a TPS is that this policy rewards innovative firms for emissions reductions because reducing emissions generates compliance credits that can be sold to firms whose performance would otherwise fall short of the standard.
A second advantage is that a TPS is less likely than a carbon price to harm industrial sector employment or cause emissions leakage. (Leakage happens when a regulation in one jurisdiction raises costs enough to shift economic activity and corollary emissions from a regulated area to an unregulated area.) The emissions leakage and adverse employment effects that do occur depend on the magnitude of the cost increase caused by regulation. A TPS raises costs only for businesses that use inputs with higher emissions intensity than the industry benchmark. Consequently, a TPS causes a smaller overall cost increase by reducing emissions leakage and employment losses, compared to other potential policies such as a carbon price.
A key disadvantage of a TPS, compared to a carbon price, is that the smaller product price increases provide less incentive for consumers to substitute shift away from emissions-intensive products and toward more environmentally friendly products. Applying multiple industry- or product- specific standards can further increase costs.
Key Considerations
Recent research reveals large differences between the highest and lowest energy-intensive production facilities within various industrial subsectors, including cement, bulk chemicals, and iron and steel. This suggests that potentially large efficiency gains may be attainable, and a TPS is a plausible mechanism for achieving these gains.
One of the primary factors policymakers must consider when designing a TPS is how to manage the diversity of products and industries within the sector. The complexity can pose a challenge, particularly for subsectors with a wide variety of products, whereas establishing benchmarks for products that are relatively homogeneous—such as some cement products, basic steel, and bulk chemicals—may be less challenging. Strategies to allocate emissions allowances in cap-and-trade programs can be adapted to define TPSs for heterogeneous subsectors.
Past, Current, and Proposed Tradable Performance Standards
To date, application of TPSs to industry has been limited. A proposal by Representative Sean Casten (D-IL) would cover both the industrial sector and the electricity sector. The proposal would apply a clean energy standard to electricity generators and industrial thermal energy generators, requiring that energy producers meet certain emissions-intensity benchmarks for the energy they generate.
Another example of a TPS that spans sectors is California’s Low Carbon Fuel Standard. This policy regulates the carbon intensity of the full life cycle of transportation fuels, incentivizing carbon reductions in transportation, electricity, and the agricultural activities that fuel transportation.
China also has announced its intention to establish a TPS for the nation’s power sector and various industrial sectors.
Research, Development, and Demonstration
The Basics
Government funding for carbon-reducing technologies can help technologies mature, become less costly, and reach commercial scale. Research, development, and demonstration (RD&D) is particularly important for reducing industrial emissions, as some current industrial production processes offer few opportunities to reduce emissions.
Many policy options are available to help fund innovation. These policies can be classified as “supply-push” and “demand-pull” policies. Supply-push policies are traditional ways of issuing government-funded grants, such as ManufacturingUSA, or funding for technology development at federal laboratories. Demand-pull policies aim to shape or stimulate the market for technologies by creating demand or reducing uncertainty in prices. Demand-pull policies include government green procurement policies; contracting guidance; market-creation mandates; and prizes, challenges, or milestone programs that better target RD&D.
Benefits and Challenges
RD&D funding can lead to the commercial viability of advanced energy technologies and emissions-reducing technologies and it can also enable the discovery of new technologies. These technological advances and discoveries could both reduce the industrial sector’s carbon footprint and make emissions reductions less costly as more technologies compete to provide reduced-carbon (or carbon-free) products.
The primary drawback to funding RD&D is that, of course, the government must pay for it, which drains government revenues. Additionally, there is inherent risk in RD&D spending, as the efforts do not guarantee technological advances. That said, policies can be designed to minimize such risks.
Key Considerations
When allocating funds for RD&D, policymakers must work to ensure funds are spent wisely. However, it is unclear which technologies warrant the most investment, as future advances cannot be predicted. It is similarly unclear which policies will be most beneficial, which areas of technological development should be invested in the most, and which sectors will benefit most from these investments. RFF research is underway to assess the benefits of cost reductions for different advanced energy technologies.
Past, Current, and Proposed RD&D Funding
The Energy Act of 2020, which passed as part of the omnibus spending bill in December 2020, is an example of using federal RD&D spending to promote industrial decarbonization. This legislation increases funding for CCUS, direct air capture, advanced nuclear reactors, and energy storage technologies—all of which can help to decarbonize industry.
The US government supports a range of other initiatives to boost RD&D. For instance, H2@scale is an RD&D funding opportunity for cooperative agreements between the private sector and the US Department of Energy’s National Laboratories to develop and implement new technologies.
This explainer was featured in the 207th issue of Resources magazine.